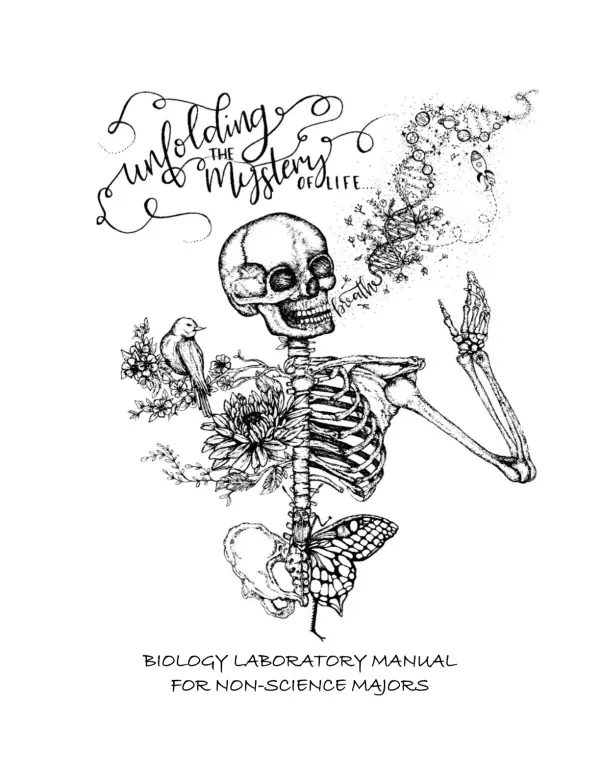
Lab Safety: Biology Manual
Document information
Author | Ellen Genovesi |
School | Mercer County Community College |
Major | Biology |
Place | West Windsor, NJ |
Document type | Laboratory Manual |
Language | English |
Format | |
Size | 1.65 MB |
Summary
I.The Metric System and Units of Measurement
This section discusses the historical context and global adoption of the metric system, now formally known as the International System of Units (SI). It highlights the standardization brought about by the 1875 Treaty of the Meter and contrasts the widespread use of the SI system in science with the continued reliance on the more complex imperial system in countries like the United States, Burma, and Liberia. Keywords: Metric system, SI units, International System of Units, units of measurement, scientific measurement, imperial system.
1.1 The Development and Adoption of the Metric System
The section begins by highlighting the historical context of the metric system's creation in 18th-century France. Prior to its development, units of measurement for length, area, and weight varied significantly between and even within countries. Length, for example, was measured using diverse units like feet, miles, spans, cubits, hands, furlongs, palms, rods, or chains. The introduction of the metric system aimed to bring order to this chaotic landscape of weights and measures prevalent in Europe at that time. The text underscores the system's success in unifying measurements, contrasting the previously confusing array of inconsistent units. This standardization significantly impacted scientific research and commerce, facilitating easier exchange and understanding of data across geographical boundaries. The significance of this standardization cannot be overstated in its contribution to the advancement of science and engineering.
1.2 The Treaty of the Meter and the International System of Units SI
A pivotal moment in the history of standardized measurement is marked by the 1875 Treaty of the Meter. This international agreement led to the formation of the International Bureau of Weights and Measures, a crucial step toward global consistency in units. The document then introduces the International System of Units (SI), the modern name for the metric system, emphasizing its global acceptance within the scientific community. The treaty's signing by most industrialized nations cemented the metric system’s importance, demonstrating the global recognition of the need for consistent measurement standards. The creation of the International Bureau highlights the collaborative effort required for the successful adoption of a universal system, showcasing an important example of international cooperation in advancing scientific progress. The bureau itself continues to play a critical role in maintaining and refining the SI system, ensuring that global measurements remain accurate and consistent.
1.3 Global Adoption and the Persistence of the Imperial System
Despite the widespread adoption of the SI system, the document notes a notable exception: the United States, along with Burma and Liberia, are identified as the only countries that don't routinely use the metric system. This fact underscores the persistent challenge in fully implementing a universally accepted measurement system. The text highlights the contrast between the scientific community's nearly universal use of the SI system and the continued use of the more complicated imperial system in daily life for many people in the United States. This observation reveals a disconnect between scientific practice and everyday life, suggesting cultural and practical factors that hinder widespread adoption. This contrast illustrates the long-term challenges involved in transitioning from established, culturally ingrained systems to new, more standardized ones. The continued use of the imperial system in these countries offers a case study for analyzing factors that affect the adoption of internationally standardized units.
II.Observation of Microorganisms Kingdom Protista
This section introduces the Kingdom Protista, focusing on single-celled eukaryotic organisms. It describes different mechanisms of motility in protists, including flagella, cilia, and pseudopods. The presence of other microorganisms like bacteria and rotifers in pond water samples is also noted. Keywords: Protista, eukaryotic cells, microorganisms, flagella, cilia, pseudopods, bacteria, rotifers, pond water.
2.6.1 Defining Kingdom Protista
This subsection introduces the Kingdom Protista, characterizing its members as single-celled eukaryotic organisms. The defining feature of these organisms is the presence of a nucleus and a complex internal cellular structure, distinguishing them from prokaryotic cells. The organisms discussed are commonly found in pond water, making it a readily available source for observation. The diverse nature of protists is highlighted, as the kingdom is divided into phyla based on the organelles responsible for their motility. This points to the diversity of adaptations within this single-celled kingdom, highlighting the evolutionary pressures that have shaped these organisms. The description establishes a foundation for understanding the complexities of even single-celled organisms and their ecological roles.
2.6.2 Mechanisms of Motility in Protists
The subsection details the various methods of movement employed by protists. It describes flagella as long, whip-like tails, cilia as shorter but more numerous structures beating like oars, and pseudopods as cytoplasmic extensions functioning as 'false feet.' Each of these structures represents a distinct evolutionary adaptation for locomotion. The comparison of flagella and cilia highlights the diversity of structures that can achieve similar functions, showcasing evolutionary convergence. The description of pseudopods, with their dynamic nature, emphasizes the plasticity of cellular structures in facilitating movement. Understanding these diverse mechanisms of movement is crucial to appreciating the ecological roles and adaptability of these organisms.
2.6.3 Associated Organisms in Pond Water Samples
Beyond protists, the subsection mentions the potential observation of other organisms in pond water samples. This includes bacteria, which represent a separate domain of life, and multicellular animals called rotifers. The inclusion of bacteria emphasizes the microbial diversity in aquatic environments, showing that pond water samples contain a complex community of organisms. The mention of rotifers, as multicellular animals, further underscores the ecological complexity of even seemingly simple habitats. The mention of these additional organisms is important for emphasizing that ecological communities are not solely comprised of a single taxonomic group but are instead a rich mix of species. Observing these organisms in a pond water sample highlights the richness and diversity of microscopic life.
III.Scientific Method and Experimental Design
This section explains the principles of the scientific method, emphasizing the roles of hypotheses, theories, and the importance of controlling experimental bias and random error. The need for controlled variables and replication in experiments is stressed to ensure reliable data. Keywords: Scientific method, hypothesis, theory, experimental error, random error, bias, controlled variables, experimental design, replication, reliable data.
3.1.1 The Nature of Science and the Scientific Method
This section establishes science as a dynamic and methodical approach to understanding the natural world. A core component of the scientific method is the hypothesis, defined as a testable explanation for an observation. The text emphasizes that hypotheses need not be correct but must be falsifiable, meaning they can be proven wrong through experimentation. This underscores the iterative and self-correcting nature of scientific inquiry, where hypotheses are refined or replaced as new data emerge. The progression from a confirmed hypothesis through rigorous testing to the establishment of a theory is clearly delineated. The explanation of theories as coherent sets of confirmed hypotheses highlights the importance of repeated experimental verification in the scientific process. The example of gravity, and the refutation of the earth-centered model of the solar system, illustrates how scientific theories evolve with the accumulation of evidence.
3.1.2 Sources of Experimental Error and Bias
The section addresses potential errors in experimentation, differentiating between random error and bias. Random error is attributed to imperfections in measuring instruments, while bias arises from a researcher's preference for a certain outcome. This discussion is crucial for understanding the need for careful experimental design and control. The distinction between these two error types highlights their differing impacts on the validity and reliability of results. The explanation emphasizes the importance of minimizing both types of errors to enhance the trustworthiness of scientific findings. The example of how bias can lead to the unconscious ignoring of data further emphasizes the need for objectivity and rigorous methodologies in research.
3.1.3 Controlled Variables Data Collection and Replication
This subsection focuses on the importance of controlled variables in experimentation. Maintaining constant conditions between experimental and control groups ensures that changes observed are attributable to the manipulated variable. The importance of this concept is emphasized by the example of investigating the effects of aspirin on heart disease, with multiple controlled variables listed. This highlights the multifaceted nature of scientific inquiry, and how complex interactions must be considered. The necessity for careful data collection through counting or measurement is also stressed, along with the importance of experimental replication to ensure the reliability and generalizability of results. This section underlines the systematic approach required for valid and reliable scientific findings, highlighting the critical role of rigorous methodologies in generating trustworthy scientific knowledge.
IV.Diffusion and Osmosis Across Cell Membranes
This section explores diffusion and osmosis as passive transport mechanisms across selectively permeable membranes. It examines the factors influencing membrane permeability and uses the example of starch and iodine to illustrate these processes. Keywords: Diffusion, osmosis, passive transport, selectively permeable membrane, cell membrane.
B.1 Membrane Permeability and Passive Transport
This section introduces the concept of how cells acquire necessary molecules and ions from their extracellular fluid. The passage of molecules across the cell membrane is influenced by factors like size, charge, and lipid solubility. Small molecules, such as water, oxygen, amino acids, and ions, easily cross the membrane via passive transport processes like diffusion and osmosis, which do not require energy expenditure by the cell. In contrast, larger or less soluble molecules require energy-consuming active transport mechanisms to cross the lipid bilayer. This fundamental difference in transport mechanisms highlights the selective nature of the cell membrane, regulating the passage of substances based on their properties and the cell's needs. The description of the lipid bilayer's role further emphasizes the selective nature of the membrane and its importance in maintaining cellular homeostasis.
B.2 Experimental Investigation using Starch and Iodine
The section outlines an experiment designed to investigate the ability of starch and iodine to cross a selectively permeable membrane. A colorimetric test is used to track the movement of these molecules. Dialysis tubing, with its microscopic pores, serves as a model of the cell membrane. The use of dialysis tubing as an experimental model provides a simplified system for studying membrane permeability. The colorimetric test allows for the visualization of molecular movement, making it an accessible and effective method for demonstrating the principles of diffusion across a membrane. The questions posed at the end of the section – identifying the smaller molecule between iodine and starch, and classifying diffusion as passive or active transport – are designed to assess understanding of the concepts discussed.
4.3 Osmosis A Special Case of Diffusion
This subsection defines osmosis as a specific type of diffusion involving the movement of water molecules across a selectively permeable membrane. Osmosis proceeds from a region of high water concentration to a region of lower water concentration until equilibrium is achieved. Larger molecules do not readily cross the membrane in osmosis. The explanation clarifies that osmosis is a passive process driven by the concentration gradient of water. The description differentiates osmosis from general diffusion by highlighting the specific role of water molecules and the selective permeability of the membrane to larger molecules. This detailed explanation provides clarity on a critical process central to cell biology and physiology.
V.Osmosis Disaccharides and Human Nutrition
This section defines osmosis and discusses disaccharides like sucrose and lactose, including the role of the enzyme lactase and lactose intolerance. It also briefly touches upon human nutrition, mentioning the importance of carbohydrates, lipids, and proteins as energy sources and building blocks. Keywords: Osmosis, disaccharides, sucrose, lactose, lactase, lactose intolerance, carbohydrates, lipids, proteins, human nutrition.
4.3.1 Osmosis and Water Movement
This subsection defines osmosis, explaining it as a specialized form of diffusion where water molecules move across a selectively permeable membrane. This movement is driven by a difference in water concentration, proceeding from an area of high water concentration to an area of low water concentration. The membrane's selective permeability ensures that larger molecules cannot easily pass, while water molecules move freely. This process continues until equilibrium is reached, meaning the water concentration is equal on both sides of the membrane. The explanation emphasizes the passive nature of osmosis, driven by the inherent tendency of water to equalize its concentration across a barrier. The focus on water as the specific molecule undergoing this type of diffusion highlights a key process for cellular function and regulation.
4.3.2 Disaccharides Sucrose and Lactose
The subsection introduces disaccharides, which are formed by the joining of two simple sugars. It provides examples such as sucrose (table sugar), formed from glucose and fructose, and lactose (milk sugar), composed of galactose and glucose. The importance of the enzyme lactase in breaking down lactose into its monosaccharide components is explained. The breakdown of lactose is necessary for proper digestion, and a deficiency in lactase leads to lactose intolerance. This detail highlights the role of specific enzymes in digestion and the impact of enzyme deficiencies on human health. The examples of common disaccharides clarify the structure and function of these important carbohydrates.
4.3.3 Human Nutrition and Biomolecules
The final part of this section provides a brief overview of human nutrition. Humans, as omnivores, consume a variety of foods from different ecosystem levels. The essential biomolecules – carbohydrates, lipids, and proteins – provide energy and building blocks for bodily functions. The mention of vitamins and minerals as important dietary components, although requiring only small amounts, completes the overview of nutritional requirements. The text emphasizes the role of these biomolecules as both energy sources and structural components in the human body. The brief overview provides a contextual framework, linking osmosis and disaccharide metabolism to overall human nutritional needs. This establishes the importance of understanding the basic processes discussed in the context of the human organism.
VI.Lipids and Proteins Structure and Function
This section describes the structure and functions of lipids (including oils, fats, and waxes) and proteins. It highlights the role of lipids in energy storage, cell membranes, and hormone production, and discusses the digestion of lipids via lipases and emulsification. It also details the structure and function of proteins, their role as enzymes, and the Biuret test for protein identification. Keywords: Lipids, proteins, enzymes, lipases, emulsification, Biuret test, amino acids, peptide bonds.
5.3 Lipids Structure Function and Digestion
This section defines lipids, encompassing oils, fats, and waxes. Their roles in energy storage, cell membrane structure, and hormone production are highlighted. The text emphasizes that dietary lipids are insoluble in water and require digestion into smaller molecules for bodily utilization. This process involves emulsification with bile salts and enzymatic breakdown by lipases in the small intestine. The description of lipids' diverse functions highlights their importance in various biological processes. The explanation of lipid digestion emphasizes the necessity of enzymatic action and the role of bile salts in facilitating the absorption of these otherwise insoluble molecules. The details regarding the location of emulsification (small intestine) provides crucial physiological context to the process.
5.4 Proteins Structure Function and Identification
This section focuses on proteins, describing them as complex, often three-dimensional molecules built from amino acid building blocks joined by peptide bonds. Proteins are essential for diverse functions including structural support of cells, hormone production (like insulin), oxygen transport (hemoglobin), muscle function, enzymatic activity, and determining characteristics such as eye and hair color. The Biuret test, which identifies proteins by changing color in the presence of peptide bonds, is mentioned as a key method of detection. The numerous functions listed underscore proteins' central role in nearly all biological processes. The description of the Biuret test provides a practical method for protein identification, linking theoretical concepts to experimental techniques. The explanation connects protein structure (amino acid sequence and three-dimensional shape) to its function, particularly the crucial role of enzyme shape in substrate interaction.
5.4.1 Enzymes Protein Catalysts
This subsection specifically discusses enzymes, emphasizing that all enzymes are proteins. The three-dimensional shape of an enzyme is critical for its interaction with its substrate (the molecule the enzyme acts upon). Enzyme action converts the substrate into products, while the enzyme itself remains unchanged and can be reused. Optimal enzyme function is dependent on specific temperature and pH conditions. The detailed explanation of enzyme function highlights their crucial catalytic role in biological reactions. The connection between enzyme shape and substrate interaction underscores the importance of protein structure in determining its function. The final point on optimal temperature and pH demonstrates the sensitivity of these crucial biological catalysts to environmental conditions.
VII.Photosynthesis and Experimental Investigation
This section explains the process of photosynthesis, dividing it into the light-dependent reaction and the Calvin cycle. It details an experiment investigating the effect of different light conditions on the rate of the light-dependent reaction, measuring oxygen production in spinach leaf disks. Keywords: Photosynthesis, light-dependent reaction, Calvin cycle, chlorophyll, oxygen production, experimental design.
6.1 Photosynthesis A Metabolic Pathway
This section describes photosynthesis not as a single reaction, but as a metabolic pathway—a series of reactions. It's divided into two phases: the light-dependent reaction and the Calvin cycle. In the light-dependent reaction, chlorophyll absorbs light energy, which is used to split water molecules. This process releases energy stored as ATP and NADPH, with oxygen released as a byproduct. The Calvin cycle utilizes the energy harvested in the light-dependent reaction to fixate carbon atoms from carbon dioxide, ultimately forming glucose. The division of photosynthesis into these two phases clarifies the distinct steps and energy transformations involved. The chemical equation provided (6CO2 + 6H2O -> C6H12O6 + 6O2) summarizes the overall process, highlighting the reactants and products. The description of energy storage as ATP and NADPH emphasizes the crucial role of these energy-carrying molecules in driving subsequent reactions.
7.2 Experimental Design Investigating the Light Dependent Reaction
This section details an experiment designed to examine how different light conditions affect the rate of the light-dependent reaction of photosynthesis. The rate is estimated by measuring oxygen production in spinach leaf disks. The presence of gas-filled intracellular spaces in leaves, allowing leaves to float on water, is noted. Leaf disks are treated with sodium bicarbonate solution and subjected to a vacuum to remove trapped gases, causing them to sink. The disks are then exposed to different light conditions (dark, room light, and sunlamp) and observed for oxygen production, measured by the number of floating disks after 20 minutes. The experimental design includes careful procedures to control variables and ensure consistency. The method of measuring oxygen production, based on the buoyancy of the leaf disks, is both simple and effective for demonstrating the principles involved. The use of a control group (dark condition) is implied and is crucial for interpreting the results.
7.2.1 Step by Step Procedure for Photosynthesis Experiment
This subsection provides a detailed, step-by-step procedure for conducting the photosynthesis experiment. It involves cutting spinach leaf disks, placing them in a sodium bicarbonate solution, removing trapped gases using a vacuum, and then exposing the disks to various light conditions (dark, room light, and under a sunlamp). After a 20-minute period, the number of floating disks in each treatment group is counted to measure the rate of oxygen production. The precise steps outlined in this subsection ensure reproducibility of the experiment. The inclusion of specific details such as the number of leaf disks (60), the use of a core borer, and instructions to avoid large leaf veins highlights the attention to detail necessary in conducting scientific investigations. The instructions for data recording and analysis (creating a bar graph) provide guidance for interpreting the results.
VIII.Human Genetics and Karyotypes
This section covers human genetics, discussing the number of chromosomes in human cells (46), the concept of genes, and the use of karyotypes to represent an individual's chromosomes. Keywords: Human genetics, chromosomes, genes, karyotype.
8.2 Human Chromosomes and Genes
This section begins by stating that each human somatic cell contains 46 chromosomes within its nucleus. Each chromosome, in turn, carries thousands of individual genes, with an estimated 20,000 genes in the human genome. Interestingly, the text points out that the number of genes in humans is not significantly different from that found in chimpanzees or mice. This observation highlights the complexity of the human genome and the shared genetic heritage among different species. The information emphasizes the vast number of genes within a single cell, each carrying specific genetic instructions. The comparison with other species provides a broader evolutionary perspective, suggesting that significant biological complexity can be achieved with a relatively similar number of genes.
8.2.1 Karyotype Analysis
The subsection describes the process of karyotype analysis, beginning with the extraction of chromosomes from cells. These chromosomes are then prepared on a glass slide, stained with dye, and examined under a microscope. Each chromosome pair is assigned a number (1 to 22, then X and Y) based on its staining pattern and length. A karyotype is defined as a visual representation of a person's chromosomes, shown in pairs and arranged in decreasing size. While a karyotype reveals the overall chromosomal structure, individual genes cannot be seen at this level of resolution. This description details the process of creating a karyotype, a fundamental tool in cytogenetics. The limitation of karyotype analysis – the inability to visualize individual genes – highlights the need for more advanced techniques for studying specific genes and their functions.
8.2.2 Case Study Karyotype 3
The subsection presents a case study involving Karyotype 3 from a handout (not included here). An 11-year-old patient is described as having developmental delays in language skills, inattention, and hyperactivity. The patient's height is at the 85th percentile, while weight is at the 45th percentile. This case study provides a practical application of karyotype analysis in diagnosing potential genetic abnormalities. The information about the patient's developmental delays, behavioral characteristics, and unusual height-to-weight ratio suggests a possible link to a genetic condition. The reader is prompted to consider potential causes for the described abnormalities, emphasizing the importance of applying karyotype analysis to understanding and diagnosing clinical conditions.
IX.Genetic Crosses and Stickleback Fish
This section introduces the concept of genetic crosses using stickleback fish as an example to study the inheritance of phenotypes and determine whether traits are dominant or recessive. Keywords: Genetic crosses, phenotypes, dominant, recessive, alleles.
9.1 Introduction to Genetic Crosses
This section introduces the field of genetics and its methods for understanding inheritance. Geneticists employ breeding experiments, known as genetic crosses, to investigate how traits are inherited. The primary goal is to determine if traits are inherited through dominant and recessive patterns, and whether a single gene or multiple genes are involved in controlling a particular phenotype. This section establishes the fundamental approach of genetic crosses as a method for understanding inheritance patterns. The questions posed – about dominant vs. recessive traits and the number of genes involved – highlight the key questions that genetic crosses aim to answer. The mention of Dr. David Kingsley's work in the film 'Evolving Switches, Evolving Bodies' provides a real-world example of the application of genetic crosses in research.
9.1.1 Stickleback Fish as a Model Organism
The section focuses on using stickleback fish in a genetic cross experiment to study the inheritance of pelvic spines. This involves breeding stickleback fish with pelvic spines with those lacking pelvic spines to observe inheritance patterns. The specific choice of stickleback fish implies a pre-existing knowledge base about the genetics of this organism. The contrasting traits (presence or absence of pelvic spines) are clearly defined, providing a simple yet effective system for studying inheritance. The use of this organism as a model system demonstrates how particular species can provide valuable insights into fundamental biological mechanisms. This experimental design will allow researchers to analyze inheritance patterns and determine whether the presence or absence of spines is controlled by a single gene or multiple genes.
X.Blood Detection and DNA Fingerprinting
This section describes the Kastle-Meyer test for presumptive blood detection and the use of DNA fingerprinting in forensic science, paternity testing, and diagnosing inherited disorders. It explains the role of restriction enzymes, PCR, and gel electrophoresis in DNA analysis. Keywords: Kastle-Meyer test, DNA fingerprinting, restriction enzymes, PCR, gel electrophoresis.
10.1 Blood Detection using the Kastle Meyer Test
This section describes the Kastle-Meyer test, a rapid and inexpensive presumptive test for blood. The test uses phenolphthalein, which reacts with hydrogen peroxide in the presence of hemoglobin (the oxygen-carrying protein in blood) to produce a color change from colorless to pink. The test is considered presumptive because other substances, such as certain foods containing hemoglobin or some vegetables, can also yield a positive result. However, the advantage is that the tested sample remains intact for further analysis. The simplicity and speed of the Kastle-Meyer test make it a valuable tool for initial screening at crime scenes or in other situations where rapid blood detection is needed. The caveat of false positives highlights the importance of using this test as a first step, followed by confirmatory testing to ensure accurate identification.
10.2 DNA Fingerprinting Techniques and Applications
The section introduces DNA fingerprinting, a widely used technique in forensic science, paternity testing, and diagnosing inherited disorders. It's used to determine whether two DNA samples originate from the same individual. The process involves using restriction enzymes to cut DNA molecules at specific sites, creating fragments of varying lengths. These fragments are then separated using gel electrophoresis. PCR (polymerase chain reaction) can amplify small or degraded samples, making analysis possible even with trace amounts of DNA. The separated fragments are identified using radioactive markers, producing a pattern of lines that resembles a barcode, forming the DNA fingerprint. The application of DNA fingerprinting in various fields, including forensics, highlights its power in providing conclusive evidence. The description of the techniques, including restriction enzymes, gel electrophoresis, and PCR, provides insight into the technological underpinnings of this crucial method. The use of dye mixtures to simulate DNA migration in the described experiment offers a practical and safe way to understand this complex technique.
10.2.1 Gel Electrophoresis in DNA Fingerprinting
This subsection details the gel electrophoresis step in DNA fingerprinting. After DNA samples are cut with restriction enzymes, the resulting fragments are separated using this technique. Smaller fragments move faster through the gel than larger ones, resulting in a pattern of separated bands. In the described experiment, simulated DNA samples (dyes) are used to mimic the migration of actual DNA fragments. The step-by-step procedure for preparing samples and running gel electrophoresis is outlined, including instructions for sample loading. The description of gel electrophoresis as a method for separating DNA fragments by size provides crucial information about this technique’s importance in DNA analysis. The use of simulated samples allows for a safe and simplified introduction to this crucial method of DNA analysis without the complexities and safety requirements involved in using real DNA.
XI.DNA Isolation and Cellular Structures
This section focuses on DNA isolation, particularly from plants (wheat germ and strawberries), and provides background information on the structure and function of DNA. It also briefly describes the different types of animal tissue, including muscle tissue and nervous tissue. Keywords: DNA isolation, DNA structure, eukaryotic cells, muscle tissue, nervous tissue.
11.1 DNA The Blueprint of Life
This section introduces deoxyribonucleic acid (DNA), its location in the nucleus of eukaryotic cells, and its role in directing protein synthesis. DNA is described as a polymer composed of nucleotide bases (guanine, adenine, thymine, and cytosine), and two sugar/phosphate backbones forming a double helix. The human genome comprises approximately 3 billion base pairs located on 46 chromosomes. The Human Genome Project's achievement in determining the order of nucleotides on each chromosome is mentioned. A gene is defined as a sequence of nucleotide bases that codes for a specific protein, highlighting DNA's crucial role in determining an organism's characteristics and functions. The immense number of base pairs (3 billion) within the human genome emphasizes the complexity of genetic information encoded in each cell. The mention of the Human Genome Project underscores a significant milestone in biological research, providing a comprehensive understanding of the human genetic blueprint.
11.2 DNA Isolation from Plants Wheat Germ and Strawberries
This section describes procedures for isolating DNA from plant sources: wheat germ and strawberries. Wheat germ, the embryo of the wheat seed, is noted as a rich source of DNA. The text explains that when wheat is milled into white flour, the germ and bran are removed, with whole wheat flour containing all parts of the seed. Strawberries, particularly the Fragaria ananassa variety (octoploid), are also mentioned as excellent sources of DNA, due to their high number of chromosomes (eight sets). The choice of wheat germ and strawberries highlights readily accessible plant sources for DNA extraction. The description of octoploid strawberries underscores how some plant species' higher chromosome counts result in a greater abundance of DNA. This suggests these organisms are good choices for DNA isolation exercises, enabling easier extraction.
11.2.1 Animal Tissues Muscle and Nervous Tissue
The subsection briefly describes muscle tissue, its role in movement through contraction (voluntary and involuntary muscles), and examples of its functions. It also describes nervous tissue, its role in conducting electrical impulses, and the function of neurons in receiving, processing, and transmitting impulses. Specific examples of muscle tissue functions (running, facial expressions, blood vessel diameter control) and nervous tissue components (optic nerve, retina) are given. The short descriptions of muscle and nervous tissues serve as a comparison and contrast with the earlier discussion of plant sources for DNA isolation. The inclusion of specific examples of tissue types and their associated functions within the broader context of cellular structures aids in understanding the diversity of functions that cellular components fulfill.
XII.Evolution of Mammalian Red Blood Cells and Blood Typing
This section discusses the evolution of mammalian red blood cells and their lack of nuclei, relating this to oxygen levels in the Triassic period. It also includes a blood typing simulation exercise, emphasizing the importance of blood type compatibility in transfusions. Keywords: Mammalian red blood cells, blood typing, blood transfusions, evolution.
12.4 Evolution of Mammalian Red Blood Cells
This section explores the evolutionary adaptation of mammalian red blood cells, specifically the loss of their nuclei. It connects this adaptation to environmental conditions during the Triassic period, approximately 250 million years ago (MYA), when oxygen levels were significantly lower than present day levels, and even lower than in the subsequent Jurassic period. This lower oxygen environment is posited as a selective pressure favoring the loss of nuclei in red blood cells. The loss of nuclei is presented as an evolutionary advantage under conditions of low oxygen, although the specific mechanism is not explained. The timeframe provided (Triassic period, 250 MYA) places this evolutionary event within a specific geological and environmental context. The evolutionary context emphasizes the relationship between environmental conditions and the adaptation of biological structures for enhanced survival and efficiency.
ACTIVITY Blood Typing Simulation
This section describes a blood typing simulation exercise, emphasizing the importance of blood type compatibility in blood transfusions. The scenario presented involves a technician in a city hospital needing to determine the blood types of a car accident victim and three potential blood donors to ensure safe transfusion. No actual blood products are used in this exercise. The simulation's practical nature emphasizes the importance of understanding blood typing in real-world medical contexts. The emphasis on blood type compatibility highlights a crucial aspect of safe medical practice. The mention of a 'Blood Typing Game' suggests that the simulation exercise could be made more interactive and engaging.
XIII.Microbiology Food Microbiology and Disease Transmission
This section covers microbiology, focusing on bacteria and fungi. It discusses the role of microorganisms in food production (e.g., fermentation of yogurt and cheese) and the health hazards associated with foodborne pathogens such as E. coli, Listeria, and Salmonella. It also introduces the principles of epidemiology and disease transmission pathways. Keywords: Microbiology, bacteria, fungi, food microbiology, fermentation, foodborne illness, E. coli, Listeria, Salmonella, epidemiology, disease transmission.
13.1 Introduction to Microbiology and Food Microbiology
This section introduces the field of microbiology, focusing on bacteria and fungi. Bacteria, single-celled prokaryotic organisms, are described as the most numerous and diverse organisms on Earth, inhabiting diverse environments including soil, air, water, and the human body. The text highlights that most bacteria are not pathogenic, giving examples of beneficial bacteria such as E. coli in the human intestine and Acidophilus in yogurt. Fungi, eukaryotic organisms, are also introduced, noting that most are harmless. The ubiquity of bacteria is emphasized, highlighting their presence in nearly every environment. The examples of beneficial bacteria in yogurt and the human gut contrast with the potential for harmful bacteria, setting the stage for a discussion of food safety and disease transmission. The introduction of fungi broadens the scope of the section to include a wider range of microorganisms.
13.4 Food Microbiology and Fermentation
This section focuses on the use of microorganisms in food production and preservation, citing examples that date back thousands of years (yogurt). It emphasizes that microorganisms are used to improve the taste and texture of food, citing examples like wine, beer, sausage, sauerkraut, kimchi, bread, and cheese. The industrial use of microorganisms in food production, beginning in the late 19th century, is also mentioned. The historical perspective on food fermentation highlights the long-standing relationship between humans and microorganisms. The wide variety of foods produced using microorganisms (yogurt, wine, beer, etc.) illustrates the significant impact of these organisms on human culture and diet. The text explicitly connects the historical context of food microbiology to modern industrial food production.
13.4.1 Bacteria and Health Hazards in Food
This subsection addresses the health hazards associated with harmful microorganisms in food. It stresses that even bacteria normally present in the human digestive system, like E. coli, can cause severe illness or death if ingested. Specific examples of foodborne pathogens, such as Listeria monocytogenes, Staphylococcus aureus, and Salmonella species, are listed. The potential for widespread foodborne illness outbreaks is highlighted, citing a 2019 E. coli contamination of flour that resulted in 17 infected individuals across eight states. This subsection emphasizes the serious health risks associated with improper food handling and contamination. The example of the 2019 E. coli outbreak illustrates the significant public health implications of foodborne pathogens and the importance of food safety regulations.
13.4.2 Bacteria and Fermentation in Dairy Products
This subsection focuses on the role of bacteria in the production of dairy products, particularly cheese and yogurt. Lactic acid-producing bacteria are noted as aiding in the coagulation of milk protein (casein) in cheese production, and further processing with different bacteria creates distinctive tastes and aromas. The formation of holes in Swiss cheese is attributed to the production of carbon dioxide by specific bacteria. Yogurt production is explained as the fermentation of lactose by bacteria, resulting in lactic acid and acetaldehyde, which lowers the pH and causes coagulation of milk proteins into a curd. The specific role of Lactobacillus bulgaricus is mentioned. This subsection provides detail on the specific bacterial species used and the biochemical processes involved in cheese and yogurt production. The description of the various processes demonstrates the crucial role of microorganisms in creating desired characteristics in these widely consumed foods.
13.6 Disease Transmission and Epidemiology
This section addresses the diverse pathways of infectious disease transmission. It emphasizes that most microorganisms do not cause disease. For a disease to be considered infectious, the causative pathogen must be demonstrably present in ill individuals but absent in healthy individuals. The severity of an infectious disease depends on both the pathogen's virulence and the host's resistance. The section also touches upon epidemiology, defining epidemiologists as researchers investigating disease transmission, sources, geographic distribution, risk factors, and mortality rates. Different modes of transmission are briefly listed, including respiratory droplets, contaminated food and water, and direct contact. The focus on the conditions necessary to define an infectious disease emphasizes the importance of scientific rigor in establishing causality. The description of epidemiology highlights the multidisciplinary approach required to understand and address public health challenges.