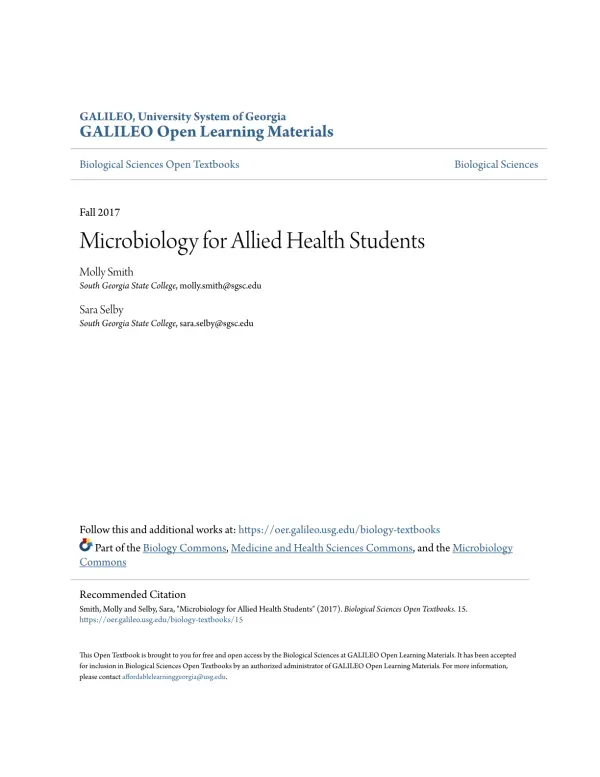
Microbiology Open Textbook
Document information
Author | Molly Smith |
School | South Georgia State College |
Major | Biological Sciences |
Document type | Open Textbook |
Language | English |
Format | |
Size | 42.89 MB |
Summary
I.The Golden Age of Microbiology and Microbial Classification
The "Golden Age of Microbiology" (1857-1914) saw breakthroughs by scientists like Louis Pasteur (fermentation, pasteurization, vaccines) and Robert Koch (linking microbes to specific diseases like anthrax, cholera, and tuberculosis). Accurate microbial classification is crucial, and Bergey's Manuals provide the standard reference for identifying and classifying prokaryotes using biochemical tests, serological tests, and DNA/rRNA sequencing due to limitations of visual identification methods. This is essential for understanding infectious disease and developing effective treatments and vaccines.
1. The Golden Age of Microbiology Key Figures and Discoveries
The period between 1857 and 1914 is referred to as the "Golden Age of Microbiology," marked by significant advancements in understanding the microbial world. Louis Pasteur, a French chemist, made groundbreaking contributions by demonstrating that individual microbial strains possess unique properties and that microorganisms cause fermentation. His invention of pasteurization, a process that kills microorganisms responsible for spoilage, revolutionized food preservation. Pasteur also developed vaccines for various diseases, including rabies, impacting both animal and human health. Concurrently, Robert Koch, a German physician, established a pivotal link between a single, isolated microbe and a specific human disease. His work definitively identified the bacteria responsible for anthrax (Bacillus anthracis), cholera (Vibrio cholerae), and tuberculosis (Mycobacterium tuberculosis), fundamentally shifting the understanding of infectious diseases. These discoveries laid the foundation for modern microbiology and infectious disease control.
2. Challenges in Microbial Classification and Bergey s Manuals
The identification and classification of microbes present unique challenges. Unlike larger organisms with easily observable macroscopic features, microbes require more sophisticated techniques. Their small size and often similar appearances necessitate methods beyond simple visual inspection. To address this, a group of microbiologists developed and continuously update Bergey's Manuals, which serve as the standard references for identifying and classifying microorganisms, particularly prokaryotes. These manuals provide a structured approach for microbial classification, utilizing biochemical tests to identify species-specific chemicals and serological tests to detect antibodies that react with particular proteins. Advanced techniques like DNA and rRNA sequencing now provide even more precise methods for identifying known species and classifying newly discovered ones, significantly enhancing microbial classification accuracy and understanding.
II.Types of Microorganisms and Their Characteristics
The document explores various microorganisms, including bacteria, viruses, fungi, and protists (like protozoa). Key characteristics of each are described, highlighting their diverse roles in the environment and their potential as pathogens. Parasitic worms (helminths) are also discussed, emphasizing their impact on global health, with diseases like guinea worm disease and the widespread occurrence of nematodes like Ascaris lumbricoides. Algae, though usually not pathogenic, are highlighted for their ecological importance and toxin production in harmful algal blooms.
1. Bacteria and Their Characteristics
Bacteria are single-celled prokaryotes, lacking membrane-bound organelles. The text highlights the importance of understanding bacterial genetics and gene regulation, particularly in the context of pathogenesis. Specific examples of bacteria mentioned include Escherichia coli and Streptococcus pneumoniae, emphasizing their virulence factors and mechanisms of infection. The document also notes the use of Gram staining to differentiate between Gram-positive and Gram-negative bacteria based on cell wall structure, a crucial step in bacterial identification and treatment strategies. Furthermore, the text describes various bacterial structures and their functions, such as flagella for motility, and cytoplasmic inclusions for nutrient storage, like volutin granules, sulfur granules, gas vacuoles, and magnetosomes, highlighting their adaptations to diverse environments. The role of teichoic acids in Gram-positive bacteria, such as Streptococcus, in binding to host cells to enhance infection is also discussed.
2. Viruses Structure Life Cycles and Diagnostic Methods
The document introduces viruses as obligate intracellular parasites, meaning they require a host cell for replication. It details the discovery of viruses, tracing back to the identification of the tobacco mosaic virus (TMV). The text then describes viral life cycles, differentiating between the lytic and lysogenic cycles in bacteriophages. The lytic cycle involves viral replication and host cell lysis, while the lysogenic cycle involves viral genome integration into the host DNA. The concept of specialized transduction, a process by which phages transfer specific pieces of bacterial DNA to new host cells, is explained. Chronic infections, caused by viruses like HIV and Hepatitis C, and their persistence mechanisms are explored. Diagnostic methods like hemagglutination assays and enzyme immunoassays (EIAs) for detecting viral antigens in patient samples are also mentioned.
3. Fungi Protists and Helminths Diverse Microbial Groups
The document covers a range of eukaryotic microorganisms, starting with fungi. It discusses fungal diversity, including medically important groups like Zygomycota, Ascomycota, and Basidiomycota. The unique features of fungal cell walls (chitin) and membranes (ergosterols) are noted. Moving to protists, the document focuses on protozoa, their diverse habitats and modes of locomotion (cilia, flagella, pseudopods), and their nutritional strategies (photosynthesis, feeding on organic material). Both free-living and parasitic protozoa are addressed, highlighting their importance in food webs and their potential to cause diseases such as malaria (Plasmodium), giardiasis (Giardia), cryptosporidiosis (Cryptosporidium), toxoplasmosis (Toxoplasma gondii), and trichomoniasis (Trichomonas vaginalis). Finally, helminths, multicellular parasitic worms, are discussed, despite not being strictly microorganisms, due to their microscopic eggs and larvae. The text highlights the global impact of helminth infections, exemplified by diseases like guinea worm disease and the widespread prevalence of nematodes such as Ascaris lumbricoides, Trichuris, and hookworms (Necator americanus and Ancylostoma duodenale).
4. Algae Ecological Significance and Toxin Production
Algae, autotrophic protists, are presented as crucial components of aquatic ecosystems, responsible for a significant portion of oxygen and organic matter production. The document explains that they belong to supergroups Chromalveolata and Archaeplastida, encompassing diverse types such as dinoflagellates, diatoms, golden algae, brown algae, red algae, and green algae. While typically non-pathogenic, the text points out that certain algal species can produce toxins. Harmful algal blooms, resulting from rapid algal growth, can cause high concentrations of these toxins, leading to liver and nervous system damage in aquatic life and humans. The use of algae-derived substances like agar, agarose, and carrageenan in laboratories and food production is also mentioned.
III.Eukaryotic and Prokaryotic Cell Structures
The text contrasts the structures of eukaryotic and prokaryotic cells. Prokaryotic cells, such as bacteria, lack membrane-bound organelles, but some possess unique inclusions for nutrient storage (like volutin granules, sulfur granules, gas vacuoles, and magnetosomes). Eukaryotic cells contain organelles like mitochondria (site of aerobic cellular respiration) and utilize diverse mechanisms like endocytosis (phagocytosis and pinocytosis) for nutrient uptake and waste removal. The cytoskeleton (with its microfilaments, intermediate filaments, and microtubules) plays a key role in eukaryotic cell shape and movement. Key differences in cell walls (peptidoglycan in bacteria, chitin in fungi) and membranes (ergosterols in fungi) are noted, impacting treatment strategies with antibiotics and antifungal drugs.
1. Prokaryotic Cell Structure Unique Features and Adaptations
Prokaryotic cells, exemplified by bacteria, are characterized by their lack of membrane-bound organelles. However, they exhibit remarkable adaptations for survival in diverse environments. The text highlights the presence of cytoplasmic inclusions in some prokaryotes. These inclusions serve various functions, including nutrient storage. For example, volutin granules store polymerized inorganic phosphate crucial for metabolism and biofilm formation, while sulfur granules store elemental sulfur used in metabolism by sulfur bacteria of the genus Thiobacillus. Other inclusions, like gas vacuoles, regulate buoyancy, allowing cells to adjust their position in a water column. Magnetotactic bacteria, such as Magnetospirillum magnetotacticum, possess magnetosomes containing magnetic iron oxide or iron sulfide, enabling them to align along magnetic fields for directional movement. The text also describes carboxysomes, structures containing RuBisCO and carbonic anhydrase essential for carbon metabolism. These examples showcase the functional complexity of prokaryotic cells and their remarkable adaptations to various environments, even in the absence of membrane-bound organelles. The text specifically mentions the cell wall composition, detailing the structure of peptidoglycan in Gram-positive and Gram-negative bacteria and the additional waxy mycolic acid layer found in Mycobacteriaceae.
2. Eukaryotic Cell Structure Organelles and Processes
Eukaryotic cells, in contrast to prokaryotic cells, possess membrane-bound organelles that compartmentalize cellular functions. The mitochondria, large and complex organelles, are central to aerobic cellular respiration. The discovery that mitochondria have their own genome and 70S ribosomes supports the endosymbiotic theory, proposing that mitochondria originated from symbiotic bacteria. Eukaryotic cells utilize a variety of mechanisms for transport, including simple diffusion, facilitated diffusion, and active transport, all shared with prokaryotes. However, a unique characteristic of eukaryotic cells is their ability to perform endocytosis. This process, involving plasma membrane invagination, enables the uptake of matter. Phagocytosis, the engulfment of large particles, and pinocytosis, the uptake of dissolved materials and liquids, are two examples of endocytosis discussed in the text. The text also describes the role of the cytoskeleton (including microfilaments, intermediate filaments, and microtubules) in cell shape, movement, and other essential cellular processes like cytoplasmic streaming and muscle movement in animals. The unique features of eukaryotic cells, including the compartmentalization of organelles, endocytosis, and the sophisticated cytoskeleton are contrasted with the simpler structure of prokaryotic cells.
3. Comparing Prokaryotic and Eukaryotic Cell Structures Key Differences
The fundamental difference between prokaryotic and eukaryotic cells lies in the presence of membrane-bound organelles in eukaryotes. This leads to a significant distinction in their cellular organization and complexity. Prokaryotes, such as bacteria, lack these membrane-bound organelles, and their genetic material is located in a nucleoid region. Eukaryotes, on the other hand, have a distinct nucleus enclosing their DNA. Prokaryotes' smaller size often correlates with simpler structures, while eukaryotes are typically larger and more complex. Transport mechanisms such as simple diffusion, facilitated diffusion, and active transport are common to both cell types; however, eukaryotes have unique mechanisms such as endocytosis for ingesting matter. Furthermore, the cell walls differ; prokaryotes have peptidoglycan (except for some archaea), while eukaryotic cells such as fungi have chitin, plants have cellulose, and animal cells lack a cell wall. The structural differences between prokaryotic and eukaryotic cells directly impact their function, metabolism, and susceptibility to various treatments such as antibiotics and antifungals, reflecting the evolutionary divergence of these two cell types.
IV.Viral Life Cycles and Pathogenesis
The document details viral life cycles, differentiating between bacteriophages, plant viruses, and animal viruses. Virulent phages exhibit a lytic cycle, whereas temperate phages can integrate into the host genome (lysogeny), leading to specialized transduction—a crucial mechanism of genetic exchange in bacteria. Chronic infections, such as those caused by HIV and hepatitis C, are contrasted with acute infections. The document also discusses the unique characteristics of viroids and virusoids as subviral pathogens, mainly affecting plants. Prions, infectious proteins causing transmissible spongiform encephalopathies (TSEs), are also highlighted. Diagnostic methods for viral infections, including hemagglutination assays and enzyme immunoassays (EIAs), are explained.
1. Bacteriophage Life Cycles Lytic and Lysogenic
The document details the life cycles of bacteriophages, viruses that infect bacteria. Virulent phages follow a lytic cycle, which involves five stages: attachment to specific bacterial surface receptors, entry (penetration) of the viral genome, biosynthesis of new viral components using the host cell's machinery, maturation (assembly of new virions), and release (lysis of the host cell). In contrast, temperate phages can undergo a lysogenic cycle, integrating their genome into the host's chromosome as a prophage. The prophage replicates along with the host DNA until induced to excise, entering the lytic cycle. A critical aspect of the lysogenic cycle is specialized transduction, where the excising phage may carry a segment of the host's DNA, transferring it to a new host upon subsequent infection. This mechanism contributes to bacterial genetic diversity and evolution. The specific examples of the T-even phage for the lytic cycle and the mechanism of specialized transduction are described.
2. Plant and Animal Virus Infections Diverse Pathogenesis
Plant viruses are described as biotrophic parasites, establishing infections without necessarily killing the host, resembling the lysogenic cycle in bacteriophages. Their life cycle involves penetration, uncoating within the host cytoplasm, replication of the viral genome, synthesis of viral proteins, assembly of new virions, and transmission to new plants. Animal virus infections are also discussed, highlighting the distinction between acute and chronic infections. Chronic infections, such as those caused by HIV and Hepatitis C, can persist for extended periods, often with prolonged latency, through several mechanisms such as preventing the expression of viral antigens, altering immune cells, and rapidly changing viral antigens through mutation. These mechanisms help viruses evade detection and clearance by the immune system. The case of HIV infection progressing to AIDS is used as an illustrative example of a chronic viral infection.
3. Subviral Pathogens Viroids and Virusoids
Beyond viruses, the document also discusses subviral pathogens: viroids and virusoids. Viroids are described as small, circular, single-stranded RNA molecules that infect plants, causing significant economic losses in agriculture. Potato spindle tuber viroid (PSTV) is highlighted as an example. Virusoids, also known as satellite RNAs, are another type of subviral pathogen. Unlike viroids, virusoids require a helper virus for replication, meaning that they are non-self-replicating. They are single-stranded RNA molecules and, once released within the host cell after the helper virus enters, they replicate independently. The small size and reliance on a helper virus differentiate them from viroids and viruses. The example of subterranean clover mottle virus and its associated virusoid is discussed. The text notes the devastating impact of viroids on commercially important crops such as potatoes, tomatoes, and avocados, emphasizing the economic consequences of these subviral plant pathogens.
4. Prions Infectious Proteins
The document introduces prions as misfolded proteins that can induce misfolding in other normal proteins, leading to the formation of plaques and causing transmissible spongiform encephalopathies (TSEs). These TSEs are rare, degenerative neurological disorders affecting the brain and nervous system. The accumulation of these misfolded proteins causes the brain tissue to become sponge-like, resulting in brain damage, loss of motor coordination, and dementia, eventually leading to death. The infectious nature of these misfolded proteins and the mechanisms by which they cause such severe neurological damage are highlighted. The lack of a cure and the rapid progression of the disease are emphasized, making it a significant concern in both human and animal health.
V.Ethical Considerations in Microbiology
The text emphasizes the importance of bioethics in microbiology research and clinical practice. Examples include the unethical experiments conducted on Guatemalan citizens, highlighting the need for informed consent and institutional review boards. Ethical dilemmas regarding treatment with experimental drugs like ZMapp during the 2014 Ebola outbreak are also discussed, exploring the balance between potential benefits and risks. The use of the HeLa cell line, derived from Henrietta Lacks without her consent, serves as a cautionary example of ethical breaches in medical research.
1. Unethical Research The Guatemalan Syphilis Study
The document highlights the profoundly unethical Guatemalan syphilis study conducted in the 1940s. American researchers intentionally exposed over 1300 Guatemalan individuals—soldiers, prisoners, prostitutes, and psychiatric patients—to syphilis, gonorrhea, and chancroid without their informed consent. The study aimed to evaluate the effectiveness of penicillin and other antibiotics against these sexually transmitted diseases (STDs). The researchers employed various methods, including facilitating sexual contact with infected prostitutes and directly inoculating subjects with the disease-causing bacteria. The investigation into this experiment, completed in 2011, revealed that many subjects received inadequate or no treatment, resulting in at least 83 deaths. This egregious violation of ethical principles underscores the critical need for informed consent, respect for patient rights, and oversight by Institutional Review Boards (IRBs) in all medical research.
2. Ethical Principles in Microbiology Research and Practice
The text emphasizes the importance of ethical principles guiding microbiology studies and clinical practice. Integrity in research is paramount, requiring rigorous adherence to ethical standards. Informed consent, a cornerstone of ethical research, entails not only agreement to participate but also a clear understanding of the study's purpose and potential risks by research subjects and patients. Respect for patient rights is crucial, necessitating confidentiality and honest communication. Institutional Review Boards (IRBs) play a vital role in reviewing and approving research protocols, safeguarding the rights and welfare of participants. Similarly, humane treatment of animals in research is essential, mandating approval from Institutional Animal Care and Use Committees (IACUCs). Maintaining accurate records, fostering honest communication, and ensuring patient confidentiality are paramount in clinical practice. These ethical considerations are fundamental to responsible scientific conduct and the protection of human and animal subjects.
3. Ethical Dilemmas in Treatment The ZMapp Case During the Ebola Outbreak
The ethical complexities of using experimental treatments during public health crises are highlighted through the case of ZMapp during the 2014 Ebola outbreak. ZMapp, an unregistered drug tested in monkeys but not in humans, was administered to two American aid workers and a Spanish priest. While the aid workers recovered, the priest died. The World Health Organization (WHO) subsequently issued a report on the ethical implications of utilizing unregistered drugs in such situations. Given the high mortality rate associated with Ebola, the panel concluded that providing potentially life-saving treatments, even if unregistered, is ethical and withholding them due to safety concerns would be unethical. This scenario exemplifies the concept of "compassionate use"—using a treatment outside the standard regulatory processes—and the ethical tensions involved in balancing the urgent need for treatment with the need for rigorous safety testing.
4. The HeLa Cell Line Ethical Issues Surrounding Henrietta Lacks
The use of the HeLa cell line, derived from Henrietta Lacks's cervical cancer cells without her knowledge or consent, exemplifies ethical challenges in medical research. Henrietta Lacks's cells were taken in 1951, and they demonstrated an unprecedented ability to grow and divide indefinitely, creating an "immortal" cell line widely used in scientific research. The lack of informed consent in obtaining these cells highlights a significant ethical breach. This case raises fundamental questions about informed consent, ownership of biological materials, and the equitable distribution of benefits and risks associated with medical research. The ongoing use and impact of HeLa cells in research underline the necessity of addressing past ethical failings and ensuring future research adheres to rigorous ethical standards.