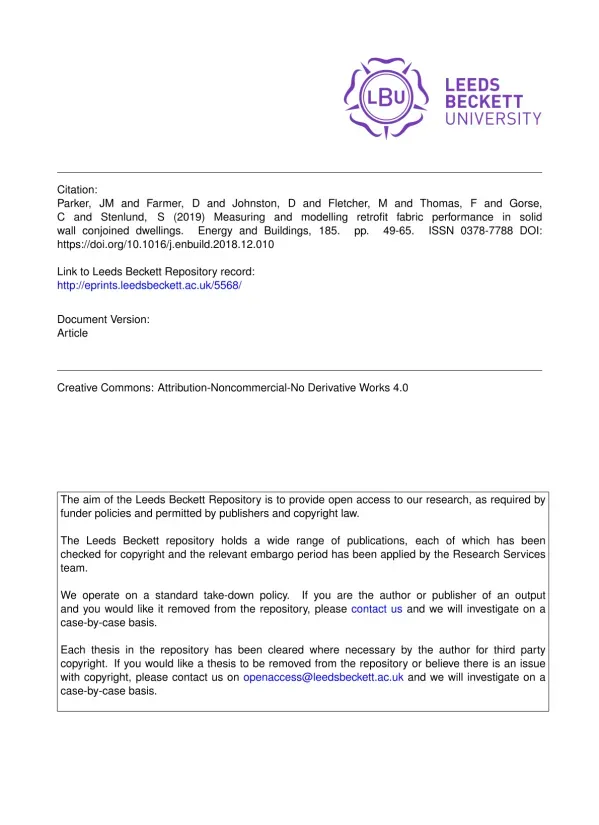
Retrofit Fabric Performance Modeling
Document information
Language | English |
Format | |
Size | 0.94 MB |
Summary
I.Domestic Energy Modelling and the Performance Gap
This section focuses on the discrepancy between predicted and actual energy performance in UK dwellings, a significant issue impacting domestic energy efficiency policy. The UK government mandates the use of the Standard Assessment Procedure (SAP) and Reduced Data SAP (RdSAP) for generating Energy Performance Certificates (EPCs). However, these simplified models often lead to a significant energy performance gap, with SAP predictions frequently overestimating energy savings. This inaccuracy poses problems for assessing the financial viability of retrofit strategies and complying with the European Directive 2002/91/EC. The use of SAP is linked to various policy instruments, including Stamp Duty exemptions and the Renewable Heat Incentive (now defunct Green Deal). The public availability of EPC data allows for meta-analysis, although the data itself is insufficient to reproduce SAP calculations.
1. The Energy Performance Gap and UK Policy
This section establishes the central problem: the significant discrepancy between predicted and actual energy performance in UK dwellings, often termed the 'energy performance gap'. This gap is a major concern for the UK's domestic energy efficiency policy. To meet the requirements of the European Directive 2002/91/EC, all dwellings for sale or rent must include an energy consumption prediction. The UK uses the government's Standard Assessment Procedure (SAP) to generate Energy Performance Certificates (EPCs), providing a benchmark for building energy performance. However, the research highlights that the SAP often overestimates absolute energy savings when compared to measured in-situ performance. This is problematic when evaluating the financial feasibility of retrofit projects and underscores the need for more accurate energy modeling.
2. SAP RdSAP and Policy Instruments
The Standard Assessment Procedure (SAP) is the cornerstone of UK domestic energy efficiency policy, primarily used to demonstrate regulatory compliance. The government's Department for Business, Energy and Industrial Strategy (BEIS) mandates the use of SAP for producing performance estimates and Energy Performance Certificates (EPCs) for dwellings. Reduced Data SAP (RdSAP) is employed for existing buildings, simplifying inputs and making several fixed assumptions. The SAP methodology is intrinsically linked to several policy instruments, including Stamp Duty exemptions for zero-carbon homes, Feed-in Tariffs, and the Renewable Heat Incentive. Importantly, SAP was also central to the now-defunct Green Deal program, with RdSAP informing economic payback calculations for retrofit measures. Publicly available data from domestic EPCs facilitates meta-analysis, though these records lack the detail needed to replicate the SAP calculations themselves.
3. Existing Building Performance Assessment Methodologies
The section explores various methods for assessing building performance, acknowledging that the performance gap isn't solely confined to SAP calculations; it also affects dynamic models used for non-domestic EPCs. The discussion covers several testing methodologies. Dynamic methods, such as the Primary and Secondary Terms Analysis and Renormalization (PSTAR) method and the electric coheating test method, are introduced. The PSTAR method, while offering dynamic analysis, presents challenges in field application due to data complexity and questioned accuracy in recent years. The coheating test, although not providing direct explanations for performance gaps, offers opportunities for additional fabric tests, such as heat flux measurements and air pressurization, valuable in identifying poor performance areas. The text also mentions quicker dynamic testing methods like Quick U-value of Buildings (QUB) and ISABELE, but notes their current limitation to research and development because of complex data analysis.
4. International Perspectives and Model Limitations
The discussion extends beyond the UK context, acknowledging that simplified energy models contribute to performance gaps internationally. While much of the existing research focuses on the UK, the authors cite numerous examples of simplified energy models causing similar performance gaps in Germany, the Netherlands, Italy, and Switzerland. The work of Sousa et al. is highlighted, evaluating 29 different models and finding them oversimplified and non-transparent, hindering comprehension of the algorithms used for energy performance prediction. However, the authors clarify that this 'non-transparency' critique doesn't fully apply to SAP, as its algorithms are publicly documented. Sousa et al. advocate for improvements in model predictions and the long-term implementation of model calibration. The influence of climate is also mentioned: in warmer climates, solar gain management and ventilation are more dominant than fabric insulation and air tightness in determining building performance; however, recent research attempts to parameterize fabric insulation’s role in overheating.
II.Testing Methodologies for Assessing Building Performance
Several methodologies exist for assessing building performance, including dynamic methods like the Primary and Secondary Terms Analysis and Renormalization (PSTAR) method and the electric coheating test method. The PSTAR method, while dynamic, involves complex data analysis, limiting its widespread application. The coheating test, though simpler, provides valuable data when combined with additional fabric tests (heat flux measurement, air pressurization) to pinpoint areas of poor thermal performance. Other dynamic testing methods, like Quick U-value of Buildings (QUB) and ISABELE, offer shorter test durations but present complex data analysis challenges, limiting their current use primarily to research.
1. Dynamic Testing Methods PSTAR and Coheating
The document introduces several testing methodologies for assessing building performance. Two dynamic methods are highlighted: the Primary and Secondary Terms Analysis and Renormalization (PSTAR) method and the electric coheating test method. The PSTAR method utilizes short-term energy monitoring (STEM) tests, such as temperature monitoring, flux measurement, and air pressurization tests, to gather performance data. This data is used as input to a model, which is extrapolated to create an annual simulation. However, the inherent complexity of the data obtained through this dynamic method can limit its practical application in the field. Concerns have also been raised regarding the accuracy of results obtained using the PSTAR method in recent years. In contrast, the electric coheating test, while not fully explaining performance gaps on its own, allows for additional fabric tests (heat flux measurement and air pressurization) to help identify areas of poor performance within a building's structure.
2. Alternative Dynamic Testing Methods and Limitations
Beyond PSTAR and the coheating test, the paper mentions other dynamic testing methods offering shorter test durations. These include the Quick U-value of Buildings (QUB) method and ISABELE (In Situ Assessment of the Building Envelope Performances). However, a key limitation of these approaches is the significantly more complex analysis required for interpreting the test data. This complexity has restricted their use mainly to research and development purposes, limiting their widespread practical application in the field. The choice of testing methodology is a critical factor in determining the accuracy of building performance assessment, and the complexity of data analysis should be weighed against the advantages of shorter test durations offered by methods like QUB and ISABELE.
III.Case Study Dwellings and Retrofit Measures
The study examines two case study dwellings built using the 1960s 'no-fines' concrete construction technique. These dwellings (Dwelling A and Dwelling B) underwent pre- and post-retrofit in situ fabric performance tests using the electric coheating test protocol. Retrofits included external wall insulation (EWI), increased loft insulation, double-glazed windows, upgraded doors, and zonal heating controls. Belfast, Northern Ireland, provided the location and relevant weather data (CIBSE TRY 2016). Detailed measurements included internal air temperatures, relative humidity, and external weather conditions (using sensors like RTDPT-100, Elster A100C kWh meters, and Vaisala WXT520). The Heat Transfer Coefficient (HTC) served as the qualifying metric for evaluating the effectiveness of the retrofit measures.
1. Case Study Dwellings No Fines Concrete Construction
The core of the study involves two case study dwellings built using the 'no-fines' concrete construction technique prevalent in the UK during the 1960s. This non-traditional method, described as a mixture of Portland cement and coarse aggregate (ratios of 1:8 to 1:10) without fine materials like sand or gravel, creates an open-textured, cellular structure. This structure results in air voids that act as insulation, giving no-fines concrete walls U-values comparable to uninsulated masonry cavity walls. However, the variability in construction practices and aggregate size and shape leads to inconsistencies in the thermal performance and quality of no-fines concrete walls. Research by Sommerville et al. highlighted the variation in void proportions within no-fines concrete, with on-site samples showing significantly higher void percentages (66%) than laboratory-cast samples (37% initially, reduced to 25% with compaction). These variations impact the overall thermal performance of the buildings, necessitating careful characterization for accurate modeling.
2. Retrofit Measures and Data Collection
Both case study dwellings underwent pre- and post-retrofit in-situ fabric performance tests. The retrofit measures implemented were designed to improve energy efficiency and included external wall insulation (EWI), increased loft insulation, double-glazed windows, upgraded doors, and zonal heating controls. The retrofit materials were chosen through a competitive tender process and evaluated by third parties as part of a wider research project. The research emphasizes that only pre- and post-retrofit SAP calculations were used in the wider project; dynamic models were developed specifically for this paper. To gather detailed data during the electric coheating tests, various parameters were monitored, including total electrical energy input, internal air temperatures and relative humidity, and local weather conditions. Specific sensors and meters used are documented, including RTDPT-100 temperature sensors, Elster A100C kWh meters, and a Vaisala WXT520 weather transmitter. Heat flux plates (HFPs) were carefully placed to measure heat flux across various building elements, avoiding thermal bridges, and their positions were carefully recorded and replicated in both the pre- and post-retrofit tests. A total of 49 HFPs were used in each dwelling, including measurements for party walls. Air tightness was evaluated using blower door tests adhering to the ATTMA Technical Standard L1, and ventilation rates were approximated using the n50/20 rule of thumb, consistent with the SAP 2012 methodology. The CIBSE Test Reference Year 2016 (TRY) weather file for Belfast was used for modeling, chosen for its proximity to the building location.
IV.Modelling Conventions and Calibration Methodology
This section compares the Standard Assessment Procedure (SAP) and dynamic simulation modelling (DSM) using DesignBuilder software (with EnergyPlus as its physics engine). Key differences include SAP's steady-state monthly calculations versus DSM's hourly dynamic heat balance calculations. The DSM models, calibrated using in situ data (including measured U-values and air change rates), provided a more accurate representation of the dwellings' thermal performance. The calibration process involved adjusting model inputs to match real test conditions, notably addressing the impact of air exchange between neighboring dwellings through the porous no-fines concrete party walls. This required adjustment of the air change rate. This highlights the importance of characterizing the physical properties of existing buildings before designing retrofit strategies. The Heat Transfer Coefficient (HTC) was a key parameter for comparison. Both SAP and DSM models used calculated thermal bridging (Ψ-values) to estimate whole-house y-values.
1. Software and Modeling Approaches SAP vs. DSM
This section details the modeling approaches used, contrasting the Standard Assessment Procedure (SAP) with dynamic simulation modeling (DSM) using DesignBuilder software and its EnergyPlus physics engine. A key difference is that SAP employs steady-state monthly heat balance calculations, while DSM performs dynamic hourly heat balance calculations. Although geometry inputs are largely similar, the DSM software was configured to calculate fabric heat loss based on internal dimensions, aligning with the SAP methodology (in contrast to the typical external dimension approach in most DSM software). The handling of solar heat gain differs significantly; DSM models incorporate hourly solar gain calculations based on real-world orientation and local weather conditions, a more sophisticated approach than SAP's simplified method of designating glazing orientation in 45° increments. The SAP calculation outputs only report electricity consumed by lighting and HVAC activities, while including heat gains from equipment and occupants in the heat balance calculation. DSM models, conversely, use occupancy and operating schedules based on NCM thermal templates from SBEM calculations. These templates provide a simple way to define daily occupancy and operating patterns not included in the SAP calculations.
2. Calibration Process and Data Inputs
The section explains the calibration methodology applied to the dynamic simulation models (DSM). The calibration process uses the building's Heat Transfer Coefficient (HTC) as the qualifying metric, allowing for the successful integration of in-situ measured fabric performance data. This eliminates the need for expensive and time-consuming site-specific weather data. Key in-situ data inputs for the calibration included measured U-values, calculated and measured air change rates, and modeled thermal bridging values. The calibration process revealed a significant deviation of U-value measurements from design values which is why a careful calibration of the models was crucial, especially for the no-fines walls. A crucial aspect involved adjusting the air change rate in the models, particularly for Dwelling A, to account for air leakage through the party walls of the no-fines concrete construction. This adjustment significantly improved the accuracy of the model's HTC predictions, highlighting the importance of realistic modeling of air exchange between neighboring properties. This inter-dwelling air movement is a critical aspect to consider, particularly when conducting detailed modeling of no-fines concrete structures and especially for energy savings predictions in retrofit scenarios.
V.Results and Discussion Model Calibration and Performance Prediction
Calibration of the DSM models using the coheating test data and measured U-values resulted in significantly improved predictions of post-retrofit Heat Transfer Coefficient (HTC) values. The models accurately predicted the proportionate improvement in HTC following the retrofit, although absolute energy and cost savings predictions differed from SAP. The study found that accounting for air leakage between adjacent dwellings, a phenomenon significant in no-fines concrete construction, was crucial for accurate model calibration and improved prediction of energy savings. The results emphasize the limitations of simplified models like SAP in accurately predicting retrofit performance and the importance of using in situ data and dynamic thermal modelling for more reliable assessments. The study suggests that EWI retrofits can achieve approximately 45% improvement in HTC. The analysis revealed that the energy performance gap is significantly impacted by the assumptions made in simplified models, such as SAP.
1. Model Calibration and HTC as a Key Metric
This section presents the results of calibrating the dynamic simulation models (DSM) using the Heat Transfer Coefficient (HTC) as the primary metric. The calibration successfully utilized in-situ measured fabric performance data, eliminating the need for costly, site-specific weather data. The calibrated DSM models showed a close match between predicted and measured post-retrofit HTC values, validating the proposed calibration method. While acknowledging the limitations of a case study methodology with a small sample size, the results support the method's effectiveness and build upon previous research using similar approaches. The calibration process required adjusting various model inputs, most notably the air change rate to account for air leakage between the dwellings through the no-fines concrete party walls. This highlights the importance of accurate characterization of the building's physical properties for achieving reliable model calibration and prediction of retrofit performance. The results show a significant improvement in the accuracy of HTC prediction after calibration which ultimately impacts the overall accuracy of energy and cost saving predictions.
2. Air Leakage and its Impact on Model Accuracy
A key finding emphasizes the significant influence of air change rates on model accuracy. Analysis revealed that the initial model's air change rates deviated from actual in-situ conditions, particularly due to inter-dwelling air movement through the leaky no-fines concrete party walls. The calibration process required a significant reduction in the air change rate, particularly for Dwelling A (25% reduction in the final calibrated model), to better reflect real-world conditions. This adjustment dramatically improved the model's accuracy for Dwelling A; the predicted HTC closely matched the measured value (within the uncertainty bounds of the test method, ±9.7 W/K). For Dwelling B, the impact was less pronounced due to its end-terrace location and less party-wall area, resulting in a 10% reduction. These results highlight the importance of considering inter-dwelling air exchange when evaluating the effectiveness of retrofit measures and in improving the accuracy of energy modelling. The findings also underscore the limitations of standardized assumptions in simplified models in accurately predicting the actual performance and the associated savings.
3. Comparison of SAP and DSM Predictions Proportionate vs. Absolute Savings
The results section compares the predictions of the calibrated DSM models against those obtained using the Standard Assessment Procedure (SAP). While the proportionate improvements in HTC following retrofit were similar between SAP and DSM (around 45-50% reduction), significant differences emerged when considering absolute energy and cost savings. The absolute savings predicted by SAP were substantially higher than those predicted by the calibrated DSM models. This difference arises from several factors: SAP assumes higher solar heat gains, and the calibrated DSM models incorporate lower HTC values. The DSM models also include hourly internal heat gains during heating periods, offsetting energy consumption. These discrepancies can significantly impact payback calculations for retrofit measures, potentially resulting in payback periods much longer in reality than initially predicted by SAP. The analysis suggests that while proportionate savings estimates might be comparable between the methods for this specific case study, it’s the absolute values that hold significant implications for the accurate evaluation of retrofit cost-effectiveness.
4. Implications for Retrofit Cost Effectiveness and Policy
The overprediction of absolute energy and cost savings by SAP has major implications for building owners and operators, particularly if these predictions are used in payback calculations, as in the now-defunct Green Deal. The study provides a numerical comparison of predicted cost savings from the retrofit using both SAP and DSM models. With a hypothetical retrofit cost of £7,000 per dwelling, SAP models (both as-built and calibrated) predicted shorter payback periods compared to the DSM models. While the proportionate savings predicted by SAP were comparable to measured results, absolute savings were overestimated. Although the results are specific to the case studies in Belfast, the proportionate savings are expected to be similar elsewhere in the UK, but the absolute savings could vary depending on the local climate. The authors emphasize that the limitations of RdSAP, commonly used for existing buildings, would exacerbate the issues highlighted. These discrepancies in absolute savings highlight that financing retrofit measures based solely on SAP-derived payback periods could lead to significantly longer actual payback times, impacting project viability and the effectiveness of energy efficiency policies.
VI.Conclusions and Implications
The research demonstrated a successful calibration methodology using in-situ data and the whole-house Heat Transfer Coefficient (HTC) to accurately predict post-retrofit performance, building upon previous work in model calibration. While the case study methodology used a small sample size, the results validate this calibration approach for predicting the fabric performance of retrofitted buildings. The findings highlight the importance of considering air leakage between dwellings (particularly relevant to no-fines concrete) when modeling energy performance, and the limitations of simplified models like SAP in accurately predicting absolute energy and cost savings. The approach presented is well-suited for large-scale retrofit programs and could be extended to new-build assessments, emphasizing the need for accurate building characterization before designing and implementing retrofit strategies to bridge the energy performance gap.
1. Validation of Calibration Methodology and Future Research
The study concludes that using the building's Heat Transfer Coefficient (HTC) as the qualifying metric allows for successful calibration of dynamic simulation models (DSM) using in-situ data. This approach eliminates the need for expensive, site-specific weather data, increasing confidence in predicting retrofitted building performance. Although the research uses a case study methodology with a small sample size, it helps validate the calibration method and reinforces similar methodologies used in previous research. The authors suggest that more research is needed to further validate this methodology and its applicability, ideally incorporating a broader range of dwelling types, forms, and fabric specifications. Further investigation is warranted to enhance the accuracy of performance estimates, particularly for deep retrofit projects, by comparing SAP inputs and outputs in more detail. Despite its limitations in scope, the study serves as a reliable proof of concept, showcasing the methodology's reproducibility.
2. Implications of Air Leakage and Model Discrepancies
The results highlight the significant impact of air change rates, especially in no-fines concrete constructions, on the accuracy of building energy modeling and the energy performance gap. Air leakage between neighboring dwellings, particularly prominent in the case study's no-fines concrete party walls, played a substantial role. The calibration of the DSM model for Dwelling A depended on incorporating a reduced air change rate (25%) to account for this leakage, which is a factor not typically accounted for in standardized assessment methods. This finding underscores the need for a more comprehensive understanding of this inter-dwelling air movement and its influence on heat loss and subsequent energy savings. This research suggests that the overestimation of absolute energy savings by simplified models like SAP, even when they incorporate higher heat gain values, is further exacerbated by unaccounted-for air leakage. The implications are substantial when considering retrofit project financing, where predicted payback periods can be significantly longer in reality than initial estimates.
3. Broader Applicability and Policy Recommendations
The study's findings have broader implications for researchers working in model calibration and validation, contributing to the development of building energy performance assessment methods grounded in in-situ measurements. This methodology holds particular promise for large-scale retrofit programs and could even be extended to calculate HTCs for new buildings. Measuring U-values more rapidly in the future could further enhance the efficiency and applicability of this approach to large new-build developments. The presented methodology allows for more accurate predictions of building performance compared to simplified models, reducing the energy performance gap. At a governmental level, such an approach could underpin policy implementation for large-scale retrofitting programs, maximizing long-term savings and cost-effectiveness for all stakeholders. However, it’s acknowledged that this approach needs further investigation across a wider range of building types and characteristics to determine the extent of its effectiveness and transferability.