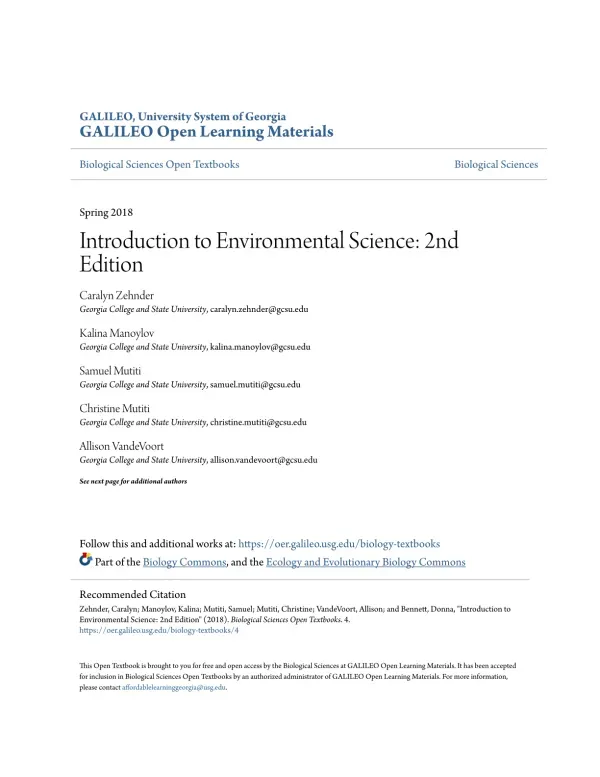
Environmental Science Open Textbook
Document information
Author | Caralyn Zehnder |
School | Georgia College and State University |
Major | Biological Sciences |
Document type | Open Textbook |
Language | English |
Format | |
Size | 25.89 MB |
Summary
I.Ecosystems and Environmental Variables
Ecosystems are complex systems where organisms compete for limited resources like organic matter, sunlight, and mineral nutrients. Natural selection drives this competition. Key environmental variables impacting ecosystem dynamics include latitude, rainfall, topography, and available species. Ecosystem size varies greatly, from small tidal pools to vast rainforests like the Amazon. Human activities, including agriculture, pollution (air pollution, acid rain), deforestation, and overfishing, significantly impact ecosystem health and the crucial ecosystem services they provide, such as water purification and coastal protection. The example of Georgia's coastal salt marshes highlights the importance of conservation efforts.
1. Competition for Resources and Natural Selection
Life within ecosystems centers around competition for limited resources. This competition, a key aspect of natural selection, occurs both within and between species. Organisms vie for resources such as organic matter (from living or deceased organisms), sunlight, and mineral nutrients—essential for energy production and the construction of physical structures. The excerpt emphasizes that this competition is a fundamental characteristic of life in any ecosystem, driving adaptation and evolution through the process of natural selection. The struggle for existence, in the context of limited resources and the resulting competition, dictates which organisms are more successful at survival and reproduction. This is central to the concept of fitness in evolutionary biology. The text does not delve deeply into specific examples of competitive interactions but establishes the overarching principle of competition as the driving force behind community dynamics in any given ecosystem.
2. Environmental Variables and Ecosystem Dynamics
Beyond resource competition, the physical and geographic environment significantly shapes community dynamics. Specific environmental variables, such as latitude, rainfall, topography (elevation), and the availability of certain species, act as determining factors for which organisms can successfully inhabit a particular area. These variables create distinct environmental conditions that select for organisms with specific adaptations. The text uses the examples of tidal pools and the Amazon rainforest to illustrate the wide range of ecosystems that exist, each shaped by its unique environmental conditions. The Amazon, with its high rainfall and specific geographic features, supports a vastly different array of life compared to a small tidal pool. The interaction of these environmental factors determines the distribution and abundance of species across different ecosystems globally. The section points towards how these abiotic factors define ecological niches and shape biodiversity.
3. Ecosystem Disturbances and Succession
Ecosystems are not static; they are regularly subjected to disturbances—environmental changes affecting their composition. These disturbances can stem from natural processes (e.g., forest fires ignited by lightning) or human activities (e.g., deforestation, pollution, overfishing). The text highlights the concept of ecological succession, the process by which an ecosystem recovers from a disturbance. Following a forest fire, for example, grasses, shrubs, and ultimately mature trees repopulate the affected area, restoring the ecosystem to its previous state, at least in composition. Human impacts have become equally significant to those of natural disturbances, significantly impacting the trajectory of ecosystems. The text does not go into the mechanics of succession in great depth, but it sets the stage for a broader understanding of ecosystem resilience and the consequences of human interference in natural recovery processes.
4. Ecosystem Services and Justifications for Conservation
Healthy ecosystems provide essential ecosystem services that benefit humans. The text uses Georgia's coastal salt marshes as a case study, highlighting their roles in water purification, supporting marine life, and protecting coastlines from storm surges. The importance of conservation is underscored through different justifications: utilitarian (providing direct benefits to humans), aesthetic (appreciating the beauty of nature), recreational (providing opportunities for outdoor activities), inspirational and spiritual (connecting with nature for personal well-being), and moral (believing that nature has an intrinsic right to exist). The specific example of Georgia's salt marshes is utilized to demonstrate the multitude of ways in which these ecosystems benefit human society and the broader environment, highlighting the many reasons why their protection is crucial. The various justifications presented serve to demonstrate the comprehensive value of these essential resources and argue for their protection.
II. Population Ecology Growth and Limiting Factors
In population ecology, understanding growth patterns is crucial. Exponential growth, as described by Malthus, occurs when resources are unlimited, resulting in a J-shaped curve. However, environmental factors limit growth, leading to a carrying capacity. These factors can be density-dependent (biological, impacting birth and death rates as population density changes) or density-independent (abiotic, like natural disasters). Life tables and survivorship curves (Type I, II, and III) are valuable tools for studying population dynamics and identifying vulnerable life stages. The text uses the example of elk population growth on an island to illustrate the concept of density-dependent limitations.
1. Exponential Growth and its Limits
The section introduces the concept of exponential population growth, a pattern where a population increases by a fixed percentage each year, resulting in a J-shaped curve when plotted. This rapid growth is based on the Malthusian model, which assumes unlimited resources. However, the text highlights that in reality, exponential growth is unsustainable. Eventually, environmental limitations, such as resource scarcity or disease outbreaks, curb population growth as the population approaches its carrying capacity and density increases. An illustrative example involves an elk population introduced to an island; initial rapid growth is eventually slowed due to factors like reduced food availability and increased disease transmission resulting from the higher density. This example showcases how environmental factors are density-dependent, influencing birth and death rates to ultimately affect population size. The concept of carrying capacity, the maximum population size an environment can support sustainably, is implied but not explicitly defined in this section. The explanation lays a foundation for the further exploration of population regulating mechanisms.
2. Density Dependent and Density Independent Factors
The text distinguishes between density-dependent and density-independent factors that influence population growth. Density-dependent factors are biological interactions, such as competition for resources, predation, or disease, whose impact on birth and death rates intensifies with increasing population density. Conversely, density-independent factors, primarily abiotic, such as natural disasters (e.g., earthquakes, floods, fires), have a similar effect regardless of population density. The passage emphasizes the distinction between the impact of these two types of limiting factors. Density-dependent factors often lead to negative feedback loops, slowing down population growth as density increases. Density-independent factors, however, can drastically reduce population sizes irrespective of existing population density. The text uses the example of an elk population on an island to illustrate how density increases affect birth and death rates, highlighting the importance of considering both density-dependent and -independent limitations to population growth. The reader is prompted to consider other examples of both types of limiting factors.
3. Life Tables and Survivorship Curves
Population ecologists utilize life tables to analyze population dynamics and identify vulnerable life stages. Life tables record survivorship—the probability of an individual surviving to different ages within a population. This approach was initially developed by the insurance industry and adapted for ecological studies. Life tables are used to construct survivorship curves, graphs depicting the proportion of individuals alive at various ages. These curves typically fall into three types: Type I (high survivorship until old age, then rapid decline), Type II (constant survivorship throughout life), and Type III (high mortality early in life, followed by higher survivorship for those who survive the early stages). The text provides examples: humans exhibit Type I survivorship, while many insects represent Type III. Analysis of survivorship curves offers insights into a population's life history strategies and the relative risks of mortality across different life stages. The information provided highlights the significant value of life tables and survivorship curves in ecological research and conservation efforts by helping identify vulnerable populations or life stages to prioritize interventions.
III.The Carbon Cycle and Human Impact
The carbon cycle describes the continuous movement of carbon through various reservoirs (pools) via fluxes. Key reservoirs include biomass (plants and animals), soil, rocks, water bodies, and the atmosphere. Human activities significantly alter the carbon cycle, particularly through the combustion of fossil fuels (coal, natural gas, petroleum) and biomass (wood, dung), releasing large amounts of CO2 into the atmosphere. Deforestation reduces carbon uptake via photosynthesis. The resulting increase in atmospheric CO2 is a major driver of climate change. The text contrasts the relatively short residence time of carbon in living organisms with the extremely long residence time in fossil fuel reservoirs (300-400 million years).
1. The Carbon Cycle Reservoirs and Fluxes
The carbon cycle is a fundamental biogeochemical process involving the continuous movement of carbon atoms through various reservoirs or pools. These reservoirs include the atmosphere, oceans, biomass (living organisms), soil, and rocks and sediments. The processes that transfer carbon between these reservoirs are known as fluxes. The text emphasizes that no new carbon is created; instead, existing carbon is continuously recycled. Carbon forms the backbone of essential biological molecules like lipids, carbohydrates, proteins, and nucleic acids, making it crucial for all life forms. A simplified illustration of the global carbon cycle is referenced (Figure 7.1), though the details of this model are not provided in the excerpt. The section's primary aim is to establish the basic structure of the carbon cycle, highlighting the concept of carbon's movement between different environmental reservoirs through various biological and physical processes. This foundation is necessary to understand the subsequent discussion of human impacts on this cycle.
2. Carbon Fluxes Respiration Consumption and Decomposition
The section details several key fluxes within the carbon cycle. Respiration, both in plants and animals, is a significant source of atmospheric carbon dioxide (CO2), as organisms release CO2 during cellular respiration. Consumption, the act of eating, moves carbon from one organism to another. Decomposers (fungi and bacteria) play a vital role by breaking down dead organic matter, releasing some carbon back to the atmosphere as CO2, and incorporating some into soil carbon. The process of decomposition varies based on oxygen availability; aerobic decomposition (with oxygen) is much faster than anaerobic decomposition (without oxygen). Anaerobic decomposition in wetland environments leads to the slow formation of fossil fuels (coal, oil, natural gas) over millions of years. The text highlights that these processes influence how carbon is stored and released, and how long it remains within each reservoir. The contrast between the rapid turnover of carbon in living organisms and the extremely slow formation of fossil fuels is particularly emphasized.
3. Human Impacts on the Carbon Cycle
The text emphasizes that human activities have significantly altered the global carbon cycle, particularly since the Industrial Revolution. The increase in the combustion of fossil fuels for energy production and transportation has drastically increased atmospheric CO2 concentrations. Land-use changes, primarily deforestation, further contribute to this increase by reducing the amount of carbon sequestered by plants through photosynthesis. The burning of biomass, both for domestic purposes (heating, cooking) and agricultural practices (slash-and-burn agriculture), also adds significant amounts of CO2 to the atmosphere. The text notes that the increased atmospheric CO2 is partly responsible for the increased CO2 dissolution in the ocean. A figure (Figure 7.1) is referenced to quantify human impacts, showing that increased CO2 emission from fossil fuel combustion and land-use changes are the most significant contributors to the current imbalance in the global carbon cycle, ultimately affecting climate change. The text also references a video demonstrating the change in atmospheric CO2 concentration over time. The text explicitly points out the impact of CO2 increases due to deforestation, fossil fuel use, and biomass burning.
IV.Atmospheric Composition and Air Quality
The atmosphere is layered (troposphere, stratosphere, mesosphere, thermosphere). Its composition is dominated by nitrogen (78%), oxygen (21%), and trace gases. Ozone (O3) plays a critical role, absorbing harmful UV radiation in the stratosphere (the ozone layer). However, ground-level ozone is a pollutant. Human-made chemicals like chlorofluorocarbons (CFCs) have caused ozone depletion, particularly over Antarctica (the ozone hole). The Montreal Protocol is an international agreement designed to phase out ozone-depleting substances. The text also discusses other air pollutants like VOCs (volatile organic compounds) and radon, highlighting their sources and health impacts. Acid rain, formed from sulfur dioxide and nitrogen oxides, is another significant air pollution problem linked to fossil fuel combustion. The Clean Air Act is mentioned as a key piece of legislation aimed at improving air quality.
1. Atmospheric Composition and Structure
The Earth's atmosphere is a layered system of gases held in place by gravity. While numerous gases are present, the top four—nitrogen (78%), oxygen (21%), argon, and carbon dioxide—constitute 99.998% of clean, dry air (excluding water vapor). The text highlights the roles of these gases: nitrogen dilutes oxygen, preventing rapid combustion; oxygen is essential for respiration and combustion; argon is inert, used in various applications; and carbon dioxide is crucial for photosynthesis and acts as a heat-trapping agent. The significance of the atmospheric composition is tied to life support and various industrial/scientific applications. While water vapor is not a main constituent in dry air, it's also acknowledged that the atmosphere is rarely completely dry. The text emphasizes that atmospheric gases obey universal physical and chemical laws and are essential for many processes both living and non-living.
2. Ozone Stratospheric Shield and Tropospheric Pollutant
Ozone (O3), a molecule consisting of three oxygen atoms, has contrasting roles. In the stratosphere, it forms the ozone layer, acting as a vital shield that absorbs most harmful UV radiation from the sun. The formation of stratospheric ozone involves the splitting of oxygen molecules (O2) by UV radiation, with the resulting oxygen atoms (O) reacting with O2 to create O3. This ozone-oxygen cycle continuously absorbs UV radiation, protecting life on Earth from its harmful effects. However, tropospheric (ground-level) ozone is a harmful air pollutant. The text details the ozone-oxygen cycle, explaining that UV-C radiation is mostly absorbed in the upper atmosphere and does not reach Earth's surface, UV-B radiation is mostly absorbed by the ozone layer and UV-A radiation largely passes through the atmosphere, although it does have some harmful effects. The different roles of ozone, as both a protector and a pollutant, are clearly emphasized to provide a complete perspective.
3. Ozone Depletion and the Montreal Protocol
The section addresses ozone depletion, primarily caused by human-made chlorofluorocarbons (CFCs). CFCs, once used in various products like refrigerants and propellants, are exceptionally stable molecules that persist in the atmosphere for extended periods. They eventually reach the stratosphere where UV radiation breaks them down, releasing chlorine atoms that catalytically destroy ozone molecules. This process is particularly pronounced in the Antarctic during the winter, due to the formation of polar stratospheric clouds. The resulting ozone depletion is manifested as the Antarctic ozone hole. International efforts to address this problem led to the 1987 Montreal Protocol, an agreement that phased out the production of CFCs and other ozone-depleting substances. While there is evidence of reduced ozone depletion, full recovery is not anticipated immediately. The text explicitly states that the Montreal Protocol, through international cooperation, has been largely successful at limiting further ozone depletion but that the recovery will be long-term and requires continued commitment.
4. Other Air Pollutants and the Clean Air Act
The text discusses other air pollutants beyond ozone, including volatile organic compounds (VOCs), radon, and the components of acid rain. VOCs are released from numerous household products and contribute to indoor air pollution, potentially causing sick building syndrome. Radon, a radioactive gas, can seep into buildings from the ground, posing a health risk. Acid rain, resulting from emissions of sulfur dioxide (SO2) and nitrogen oxides (NOx), primarily from fossil fuel combustion, has a pH below 5.6. In the United States, a significant portion of these emissions comes from electric power generation. The Clean Air Act is mentioned as a key piece of US legislation aiming to control air pollution through emission limits and state-level implementation plans. The text highlights multiple sources of air pollution (both indoor and outdoor), emphasizing the need for regulatory measures like the Clean Air Act to control and mitigate their impacts on human and ecosystem health. The roles of state and local governments in implementing the Clean Air Act are also touched upon, highlighting their importance in understanding local pollution sources and tailoring solutions accordingly.
V.Water Resources and the Water Cycle
The water cycle involves the continuous movement of water among various reservoirs: oceans, atmosphere, land surfaces, and groundwater. Evaporation and transpiration move water into the atmosphere, while precipitation returns it to the surface. Infiltration replenishes groundwater aquifers. Human activities, such as dam construction, impact water availability and ecosystems. The text discusses the problem of water scarcity, distinguishing between physical scarcity (actual shortage) and economic scarcity (lack of infrastructure and resources). Examples of water-stressed communities in Africa, Asia, and South America are mentioned.
1. The Water Cycle Processes and Reservoirs
The water cycle describes the continuous movement of water through various reservoirs: oceans, atmosphere, land, and groundwater. Key processes driving this cycle include evaporation (water changing from liquid to vapor, primarily from oceans), transpiration (water movement through plants from roots to leaves, then vaporizing), and precipitation (water falling from the atmosphere as rain, snow, etc.). Infiltration is the process where water penetrates the soil surface and replenishes groundwater aquifers. Groundwater can then seep back into surface water bodies (including oceans) or emerge as freshwater springs. The text traces the journey of a water molecule through the cycle, highlighting evaporation as the dominant pathway for water to enter the atmosphere. The text also mentions that approximately 90% of atmospheric moisture comes from evaporation, while the remaining 10% comes from transpiration. The section emphasizes that this cyclical movement of water is a continuous process driven by solar energy, with most of the water ultimately returning to the ocean.
2. Lakes Reservoirs and Ponds
Lakes, ponds, and reservoirs are bodies of standing water on land. Reservoirs are specifically artificial lakes formed by damming rivers. The text estimates there are over 300 million such water bodies globally, with Canada possessing around 60% of Earth's lakes. Despite lakes and rivers making up less than 1% of Earth's total water, they are a crucial source of freshwater for human use—drinking water, industry, irrigation, and hydroelectric power generation. The United States, for example, obtains more than two-thirds of its water from these sources. The text highlights the importance of these bodies of water as freshwater resources and implies that their management needs to consider both the natural processes involved in their formation and function, as well as human uses and potential impacts on their sustainability. Dam construction is mentioned as a human intervention that can create reservoirs but also lead to changes in the natural water flow and associated ecosystems.
3. Water Scarcity Physical and Economic Dimensions
Water scarcity arises when water demand exceeds supply. The text differentiates between physical scarcity (an actual shortage of water, irrespective of quality or infrastructure) and economic scarcity (a shortage due to insufficient financial resources or infrastructure to access safe water). It's estimated that around 1.2 million people worldwide experience physical water scarcity, while about 1.6 billion face economic water scarcity, mainly in less industrialized nations. The passage points out that for many regions, implementing adequate infrastructure could alleviate scarcity; however, in many less industrialized countries, the issue is a lack of political will, funding, and the physical systems to deliver reliable and safe drinking water. This often leads to a situation where a wealthy minority has access to clean water, while the majority are left without. Examples of this disparity are given, highlighting the significant social justice dimensions linked to water scarcity, particularly in certain communities across Africa, Asia, and South America. Southeast Kenya is given as a specific region experiencing severe water shortages due to inadequate infrastructure.
Document reference
- Water pollution (Hogan, C.)