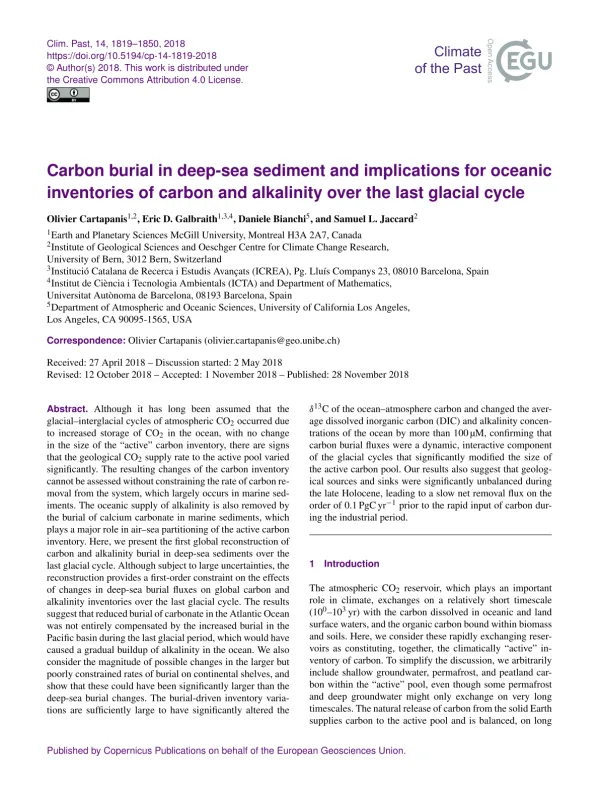
Deep-Sea Carbon Burial and Glacial Cycles
Document information
Author | Olivier Cartapanis |
School | McGill University, University of Bern, Universitat Autònoma de Barcelona, University of California Los Angeles |
Major | Earth and Planetary Sciences, Geological Sciences, Atmospheric and Oceanic Sciences |
Document type | Research Article |
Language | English |
Format | |
Size | 14.51 MB |
Summary
I.Global Carbonate Burial Reconstruction and its Impact on Glacial Cycles
This research presents the first global reconstruction of carbon and alkalinity burial in deep-sea sediments over the last glacial cycle. The study addresses the long-held assumption that glacial-interglacial cycles of atmospheric CO2 are solely due to ocean CO2 storage. Instead, it demonstrates significant variations in the geological CO2 supply rate to the active carbon pool, influencing the global carbon inventory. Reduced carbonate burial in the Atlantic, not fully offset by increased burial in the Pacific, suggests a gradual buildup of ocean alkalinity during the last glacial period. The study also highlights the substantial potential impact of changes in continental shelf burial fluxes, which could be significantly larger than deep-sea changes. The combined effects of altered deep-sea and shelf burial significantly impacted the δ13C of the ocean-atmosphere system and altered average DIC and alkalinity concentrations by more than 100 µM, confirming that carbon burial fluxes were a dynamic component of glacial cycles, modifying the size of the active carbon pool. The late Holocene shows a slow net removal flux of around 0.1 PgC yr−1 before the rapid increase during the industrial period. Key findings challenge the common assumption of a constant active carbon inventory during glacial cycles.
1. Introduction Challenging the Conventional Understanding of Glacial Interglacial CO2 Cycles
The study begins by challenging the established view that glacial-interglacial atmospheric CO2 fluctuations are solely caused by increased CO2 storage in the ocean, with a constant active carbon inventory. Evidence suggests significant variability in the geological CO2 supply rate to the active carbon pool, thereby impacting the overall carbon inventory. Accurately assessing these changes requires understanding the rate of carbon removal, primarily through marine sediment burial. The burial of calcium carbonate (CaCO3) in marine sediments is crucial, influencing the air-sea partitioning of the active carbon inventory and ocean alkalinity. The study aims to provide the first comprehensive global reconstruction of carbon and alkalinity burial in deep-sea sediments throughout the last glacial cycle to better understand these complex interactions.
2. Global Reconstruction of Carbon and Alkalinity Burial
The core of the research lies in its presentation of the first global reconstruction of carbon and alkalinity burial in deep-sea sediments during the last glacial cycle. While acknowledging substantial uncertainties, the reconstruction offers a crucial first-order constraint on how changes in deep-sea burial fluxes affect global carbon and alkalinity inventories over this period. Key findings reveal that reduced carbonate burial in the Atlantic Ocean was not fully compensated by increased burial in the Pacific. This imbalance likely caused a gradual increase in ocean alkalinity. The study further investigates potential changes in continental shelf burial rates, concluding that these could significantly exceed deep-sea burial changes. The study emphasizes that burial-driven inventory variations substantially altered the δ13C of ocean-atmosphere carbon, changing average dissolved inorganic carbon (DIC) and alkalinity concentrations by over 100 µM. This underscores the dynamic role of carbon burial fluxes in glacial cycles, modifying the active carbon pool. The late Holocene is characterized by a slow net removal flux (around 0.1 PgC yr−1) prior to the rapid carbon input from industrial activities.
3. Explaining Low Atmospheric CO2 Concentrations During Glacial Periods
The research explores proposed mechanisms to explain low atmospheric CO2 during glacial periods. Several theories suggest decreased CaCO3 burial as a contributing factor, leading to increased alkalinity and higher CO2 solubility. This decrease in burial might be due to changes in ocean circulation or biological production, increasing deep-ocean DIC and lowering deep-ocean [CO32−]. The resulting deep-ocean acidification would have necessitated CaCO3 dissolution to raise alkalinity sufficiently, rebalancing burial with alkalinity supplied by weathering. Another hypothesis proposes that reduced CaCO3 burial in coral reefs and carbonate platforms due to falling sea levels (as ice sheets grew) played a role. However, it is also argued that decreased shelf burial might have been balanced by increased deep-sea burial, thus having a limited overall effect. The study highlights the contrast between these hypotheses and the widely-held assumption of a constant active carbon inventory during glacial cycles in carbon budgeting, although some studies suggest geological carbon inputs contributed to the deglacial CO2 rise.
4. Methodology Addressing Challenges in Reconstructing Global Carbon Burial
The research acknowledges the challenges of using limited sediment core data to infer global carbon burial changes. The authors employed a three-pronged analytical strategy: (1) acknowledging the limited spatial integration of each core; (2) addressing variations between individual core accumulation rates and regional averages; and (3) considering the widespread differences in carbonate accumulation rates globally. Two related maps of modern carbonate mass accumulation rates (MAR) were used—one observationally derived and the other a metamodel—to account for spatial variability. Data from hundreds of sediment cores provided individual time series of CaCO3 MAR, used to calculate CaCO3 MAR in oceanographic provinces over time. These records reflect actual burial, not proxies. The authors account for potential biases, including issues with mass accumulation rates and sediment density, using techniques such as long-term trend correction based on a steady-state assumption between the late Holocene and MIS5e to counteract compaction effects. The study explicitly discusses potential biases and uncertainties inherent in the data and methodology.
5. Mass Balance Simulations and Their Implications
The study conducts mass-balance simulations across various scenarios to assess the impact of deep-sea and shelf burial changes on carbon and alkalinity inventories and δ13C. These simulations reveal substantial alkalinity inventory changes (up to 100 µequ kg−1) primarily influenced by deep-sea CaCO3 burial variations. Changes in deep-sea Corg burial also significantly impacted carbon inventories. The simulations encompass uncertainties in modern fluxes and investigate the effects of varied shelf burial and geological carbon input. A simplified three-box ocean model provides illustrative results for deep ocean DIC, alkalinity, and [CO32−] changes, although it omits important factors like ocean circulation and biological processes. The research underscores the importance of considering both deep-sea and shelf burial for a comprehensive understanding of glacial cycle dynamics. Importantly, only simulations incorporating variable shelf burial yield Holocene input fluxes that align with existing observational estimates.
6. Discussion and Conclusions Key Findings and Future Directions
The discussion section explores several key findings and their implications. The lack of increased deep-sea CaCO3 burial during glacial periods, despite sea-level fall and the loss of shallow-water carbonate depocenters, is discussed. This is attributed to a counterbalancing increase in deep-sea DIC. The research delves into the implications for whole-ocean δ13C and the terrestrial biosphere. Variations in CaCO3:Corg burial ratios, due to changes in shelf area, significantly impact whole-ocean δ13C, challenging its sole use as a proxy for terrestrial biosphere reconstruction. The study suggests a potentially larger terrestrial biosphere during the LGM than typically assumed. Discrepancies between simulated and observed [CO32−] changes are potentially explained by increases in soft tissue and disequilibrium DIC in the deep sea during glacial periods, consistent with findings from complex Earth system model simulations. This highlights the need for more comprehensive models incorporating these complex interactions to further refine our understanding of the carbon cycle during glacial cycles.
II.Natural Sinks and Sources of Carbon in the Climatic System
The study analyzes natural carbon sinks (organic carbon (Corg) and calcium carbonate (CaCO3) burial) and sources (geological CO2 emissions and weathering) influencing the active carbon pool (ocean, atmosphere, terrestrial biosphere). It reviews estimates of mass fluxes and carbon stable isotopic composition (δ13C) for both sources and sinks, focusing on open ocean and shallow water carbonate production and burial. Discrepancies in global Corg burial estimates highlight the incomplete understanding of coastal sedimentary environments. The paper emphasizes the importance of accurately quantifying these fluxes—including their distribution between coastal and open ocean settings and isotopic compositions—to understand past changes in global carbon and alkalinity budgets.
1. Carbon Removal Mechanisms Sinks in the Ocean
The removal of dissolved inorganic carbon (DIC) from the ocean occurs through two distinct pathways: the burial of organic carbon (Corg) and the burial of calcium carbonate (CaCO3) minerals. These processes respond differently to environmental conditions. The burial of CaCO3 plays a major role in the air-sea partitioning of the active carbon inventory and the oceanic supply of alkalinity. The paper emphasizes that understanding the rate of carbon removal from the system (largely occurring in marine sediments) is crucial for assessing changes in carbon inventories. In open ocean settings, the main CaCO3 sources are coccolithophorid and foraminiferal fragments, with burial controlled by production and export from the surface ocean and preservation at the seafloor. The CaCO3 saturation state is influenced by factors such as the concentration of Ca2+ and [CO32−], pressure, and water depth. Poorly ventilated parts of the deep ocean tend to become more acidic due to respired carbon accumulation, impacting CaCO3 preservation. Modern CaCO3 burial rates are highest in regions such as the Atlantic (due to deep CCD), eastern equatorial Pacific (high production), and southern Indian Ocean (high productivity and topography).
2. Carbonate Burial Open Ocean vs. Shallow Water
The paper contrasts carbonate burial in open ocean and shallow water environments. In shallow water settings like reefs and carbonate platforms, benthic organisms are the primary CaCO3 producers. Estimating global CaCO3 burial rates in heterogeneous coastal zones presents significant challenges. The literature reveals a wide range of estimates, reflecting the difficulty in accurately quantifying these fluxes. For example, estimates for CaCO3 buried in coral reefs during the last 6 kyr vary significantly, with values ranging from 28 PgC kyr−1 to much higher figures in earlier estimates. Similarly, estimates of the total inorganic carbon burial during the Holocene vary, highlighting inconsistencies and uncertainties in methodologies and data integration. The study mentions different approaches used to estimate these rates (e.g., focusing on coral reefs, coastal zones, or global estimates), with some studies suggesting that a substantial portion of global carbonate burial (approximately half) occurs in shallow-water environments.
3. Organic Carbon Burial A Continental Shelf Phenomenon
The paper examines organic carbon (Corg) burial, contrasting it with carbonate burial patterns. Unlike CaCO3, Corg burial is predominantly concentrated on continental shelves. This is primarily because heterotrophic organisms consume much of the organic matter in the water column, reducing sinking fluxes with increasing water depth. Conversely, carbonate minerals sink more readily. Estimates for modern Corg burial exhibit a wide range of values, differing by more than an order of magnitude across different studies (50–2600 PgC kyr−1). These discrepancies likely result from incomplete understanding of coastal sedimentary environments and differing integration times in global burial estimations on shelves. One study suggests a total global Corg burial flux of 310 PgC kyr−1, attempting to account for these uncertainties. The strong focus of Corg burial on continental margins is contrasted with relatively similar distributions of carbonate burial between deep-sea and shelf environments.
4. Isotopic Composition of Organic Carbon and Geological Carbon Sources
The study addresses the isotopic composition of organic carbon (δ13C), noting that carbon stable isotope fractionation during photosynthesis makes organic matter δ13C distinctly different from its source pool. The δ13C of modern marine surface sediment organic matter varies, reflecting multiple factors. The mean δ13C estimated from the study's database is consistent with previous literature. The second main source of geological carbon to the active pool is the transfer of carbon from exposed rocks via physical and chemical weathering. Estimates for current carbon contributions from carbonate rock weathering and petrogenic particulate Corg flux show significant variability across studies. Alkalinity fluxes from continental surfaces also impact the carbon system, with weathering of carbonate accounting for a substantial portion of the released alkalinity. The paper notes that the extent of silicate chemical weathering, physical erosion of continental surfaces, and the overall stability of global erosion across glacial-interglacial timescales are subjects of ongoing debate.
III.Analytical Strategy for Carbonate Burial Reconstruction
Due to the limited spatial coverage of sediment cores, the reconstruction utilizes a multi-step analytical strategy: (1) accounting for the limited spatial extent of each core; (2) addressing potential discrepancies between individual site accumulation rates and broader regional rates; and (3) considering variations in carbonate accumulation rates across different regions. Two modern carbonate mass accumulation rate (MAR) maps were used: one observationally based and the other derived from a process-based metamodel. The study integrates data from numerous sediment cores using province-based analysis and considers potential biases, such as variations in sediment density and mass accumulation rates. A long-term trend correction was applied using the assumption of a steady state between the late Holocene and MIS5e to minimize errors from sediment compaction.
1. The Challenge of Inferring Global Carbon Burial from Limited Data
The section begins by acknowledging the inherent limitations of using sediment cores to reconstruct global carbon burial patterns. Sediment cores only sample a tiny fraction of the global seafloor, resulting in unevenly distributed observations. To overcome this, the reconstruction must account for several key aspects: the limited spatial extent integrated by each core; the potential discrepancy between individual site accumulation rates and broader regional rates; and the significant variations in carbonate accumulation rates across different parts of the world. The uneven distribution of data and the inherent variability in accumulation rates necessitate a sophisticated analytical approach to accurately estimate global changes in carbon burial. The authors emphasize the need for a method that can effectively integrate these spatially and temporally disparate data points to provide a reliable representation of global trends.
2. Utilizing Multiple Carbonate Mass Accumulation Rate MAR Maps
To address the spatial variability in carbonate burial, the study uses two different, yet related, maps of modern carbonate MAR. The first map is observationally based, calculated by multiplying carbonate concentrations in surface sediments by the bulk sediment accumulation rate. This map leverages several existing data sources, but lacks sufficient resolution to accurately represent continental margins. The second map employs a process-based approach, integrating satellite-derived carbonate export production and water-chemistry-dependent dissolution. This metamodel, optimized to fit the sedimentary data, provides finer-grained resolution of bathymetric influences on carbonate preservation, particularly resolving continental margin features better. Using these two maps allows testing the sensitivity of the reconstructions to the choice of MAR data. While the absolute values differ, the relative changes in global carbonate burial over time are largely insensitive to this choice, strengthening the robustness of the overall findings.
3. Integrating Sediment Core Data A Province Based Approach
The core of the analytical strategy involves integrating data from numerous sediment cores. The method begins by calculating, at each 1 kyr time step over the last 150 kyr, the CaCO3 MAR in oceanographically defined provinces, expressed as a fraction of their Holocene values. This province-based approach uses individual time series from hundreds of sediment cores, incorporating CaCO3 concentrations, accumulation rates, age models, and sediment density values whenever available. The study emphasizes that these sediment cores provide records of the actual realized burial, not just proxies. While acknowledging potential biases (like underrepresentation of transient events or biases in core selection), the authors suggest that a broad spatial reconstruction minimizes locally obfuscating factors. Absolute changes in CaCO3 burial are derived by scaling the reconstructed relative changes in CaCO3 MAR to the modern MAR. Uncertainty quantification involves employing a bootstrap technique to account for variability and biases in the dataset.
4. Addressing Biases and Uncertainties Compaction and Data Coverage
The study explicitly addresses potential biases and uncertainties inherent in the data and methodology. One major source of uncertainty stems from the lack of density information for individual cores. To correct for compaction, a global-scale correction is implemented by assuming equal global bulk burial rates during the late Holocene and MIS5e and subtracting the intervening trend. This assumption, subject to future refinement, aims to minimize bias caused by compaction. The impact of mass accumulation rates and sediment density on the accuracy of burial reconstructions is explicitly discussed, with particular attention to potential biases introduced by variations in accumulation rates within cored locations. The issue of data coverage (percentage of the ocean floor represented by available cores) is also analyzed, noting that lower coverage might lead to underestimation of burial rates during certain periods, particularly older periods with fewer available data points. This emphasizes that despite its comprehensiveness, the reconstruction is likely subject to some degree of bias.
IV.Mass Balance Simulations and Implications for Carbon and Alkalinity Inventories
Mass-balance simulations using various scenarios explore the combined impact of deep-sea and shelf carbon burial changes on global carbon and alkalinity inventories and δ13C. These simulations demonstrate significant changes in alkalinity inventories (up to 100 µequ kg−1), driven by variations in deep-sea CaCO3 burial. Changes in deep-sea Corg burial also significantly altered carbon inventories. The simulations incorporate uncertainties in modern fluxes and explore the impact of varying shelf burial and geological carbon release. A three-box ocean model is used to illustrate changes in deep ocean DIC, alkalinity and [CO32−] concentrations, though these results are simplified illustrations due to the omission of circulation and biological processes. The study highlights the importance of considering both deep-sea and shelf burial fluxes for a complete understanding of glacial cycle dynamics. Notably, only scenarios that include variable shelf burial produce Holocene input fluxes consistent with observational estimates.
1. Overview of Mass Balance Simulations
The study employs mass-balance simulations to assess the impact of varying carbon and alkalinity fluxes on global inventories and δ13C. These simulations prescribe a range of plausible scenarios for variable input and output fluxes to estimate transient changes in carbon and alkalinity inventories. Deep-sea burial fluxes are derived from sedimentary reconstructions, while shelf burial fluxes and input fluxes are varied to explore a range of realistic possibilities. A total of eight scenarios are modeled to illustrate the impact of each variable individually and in combination, using a Monte Carlo approach with 1000 transient simulations per experiment. The simulations aim to quantify the influence of changes in various carbon and alkalinity fluxes on the overall system's behavior over glacial cycles. The authors acknowledge a lack of consistent data on temporally variable erosion fluxes, choosing to model erosion as constant for simplicity. This simplification is acknowledged as a limitation for future refinement.
2. Impact of Deep Sea CaCO3 Burial on Alkalinity and Carbon Inventories
The first set of simulations focuses on the impact of variable deep-sea CaCO3 burial. Assuming a constant alkalinity flux, the results suggest significant changes in the alkalinity inventory (reaching up to 100 µequ kg−1). A minimum is observed around 70 kyr (near the MIS4/MIS5 transition), followed by a gradual increase to a maximum during the early deglaciation (around 15 kyr). This is attributed to the low reconstructed carbonate burial from MIS4 to MIS2. While these simulations suggest significant changes in [CO32−], the authors note that the calculated [CO32−] changes ignore other potential influences (ocean circulation, biological processes), highlighting that the results are illustrative rather than definitively accurate. The actual changes in [CO32−] observed during the last glacial cycle are known to be much smaller, indicating that other processes besides changes in inventory are crucial in shaping the overall system.
3. Impact of Deep Sea Corg and Combined Deep Sea Burial
The analysis then examines the impact of variable deep-sea Corg burial and the combined effects of both deep-sea Corg and CaCO3 burial. Unlike CaCO3 burial, deep-sea Corg burial shows a different pattern, with larger burial during peak glacial and deglacial periods. This leads to a substantial change in the global carbon inventory (rising during MIS5 and decreasing later). The isotopic composition of the active carbon pool is also significantly affected by changes in deep-sea Corg burial, decreasing during the early glacial and showing negligible change between the LGM and late Holocene. Combining both deep-sea fluxes shows a slightly muted effect on the carbon inventory compared to considering them separately. The mean carbon input flux needed to compensate for the output fluxes in these experiments is considerably high, exceeding estimates for present-day carbon input, highlighting potential discrepancies in current carbon budget estimations.
4. Influence of Shelf Burial and the Combined Impact of Deep Sea and Shelf Burial
The study then explores the effects of variable shelf burial and the combined effect of deep-sea and shelf burial. The simulations model shelf burial changes in parallel with changes in deep-sea burial, resulting in relatively small changes in [CO32−]. However, the differing distributions of Corg and CaCO3 burial (Corg being predominantly in shallow waters) mean that the global proportion of Corg to carbonate burial varied with changing shelf area. The calculations suggest that reduced Corg burial during glacials could have lowered the δ13C of the active carbon pool. The large uncertainties in modern burial fluxes translate to significant uncertainties in the ratio of DIC:ALK changes. Notably, only simulations incorporating variable shelf burial lead to Holocene input fluxes that agree with observational estimates, indicating the crucial role of shelf burial in balancing the long-term carbon budget. The simulations reveal the complex interplay between deep-sea and shelf burial fluxes in shaping global carbon and alkalinity inventories and their isotopic signatures.
5. Incorporating Volcanic Carbon Input Variations
The final set of simulations incorporates the potential for varying carbon input from volcanic sources. Some studies suggest that volcanic carbon input increased during glacial maxima and/or deglaciations. However, the magnitude of these changes remains highly uncertain. The simulations consider a range of possible volcanic carbon input scenarios. When deep burial variations are included, the magnitude of the MIS5–MIS4 [CO32−] peak is reduced. Adding shelf burial changes leads to minimal alteration of carbonate ion variations but significant changes in δ13C. This combined scenario simulates LGM ocean δ13C values closer to observed values, even without changing parameters related to the terrestrial biosphere. The results suggest a notable consistency between scenarios that include variable shelf burial and the existing estimates of modern carbon input fluxes, although further research is needed to validate these findings and refine the uncertainties.
V.Discussion of Results and Implications
The research discusses the implications of the findings, focusing on the unexpected lack of increase in deep-sea CaCO3 burial during glacial periods despite the loss of shallow-water carbonate depocenters due to sea level fall. This is attributed to a counterbalancing increase in deep-sea DIC. The study further examines the implications for whole-ocean δ13C and the terrestrial biosphere. The changes in the ratio of CaCO3:Corg burial due to varying shelf area are shown to significantly influence whole-ocean δ13C, invalidating its use as a sole indicator of terrestrial biosphere changes. The results suggest a potentially larger terrestrial biosphere during the LGM than previously assumed, though this requires further investigation. The discrepancies between simulated and observed [CO32−] changes are likely explained by increases in soft tissue and disequilibrium DIC in the deep sea during glacial periods, further supported by other complex Earth system model simulations.
1. Deep Sea Carbonate Burial Counterintuitive Glacial Behavior
The discussion section begins by addressing the seemingly counterintuitive finding that deep-sea CaCO3 burial rates did not increase during glacial periods, despite the loss of coastal carbonate depocenters due to sea-level fall. The authors discuss that this contradicts the expectation that a constant alkalinity input from weathering, coupled with a reduction in coastal alkalinity sinks, would lead to increased ocean alkalinity and consequently, higher deep-sea CaCO3 burial. They propose that the lack of increased burial is likely due to a compensating increase in deep-ocean DIC (dissolved inorganic carbon). Since [CO32−] depends on both alkalinity and DIC ([CO32−] ≈ ALK – DIC), large changes in DIC would offset the impact of alkalinity buildup on [CO32−], preventing high [CO32−] in deep water. The study's reconstruction of CaCO3 removal fluxes in deep-sea sediments suggests lower fluxes during glacial maxima compared to the Holocene, particularly during MIS4. While deglacial pulses of CaCO3 burial have been reported in certain locations, the overall deep-sea CaCO3 burial did not show a drastic peak during the last deglaciation, although post-depositional dissolution remains a possibility.
2. The Role of Shelf Burial and its Impact on δ13C
The discussion emphasizes the significant role of changes in shelf burial on the global carbon cycle. The reduced shelf area during glacial periods, resulting in decreased burial, would have increased the active carbon and oceanic alkalinity inventories. The subsequent rise in sea level and the resumption of rapid shelf burial reversed these trends. This interplay significantly altered the global ratio of CaCO3:Corg burial, leaving a clear isotopic signature (δ13C) in the active carbon inventory, particularly showing a strong likelihood for lower δ13C during MIS3-MIS2. This is because Corg burial, which removes carbon with very low δ13C, is strongly concentrated in continental margins unlike carbonate burial which is more evenly distributed. The increased Corg burial in the deep sea during glacial maxima was not enough to counter the reduced burial on shelves. This change in the balance of CaCO3:Corg burial invalidates the use of whole-ocean δ13C as a simple proxy for changes in the terrestrial biosphere, introducing considerable complexity to the interpretation of isotopic data.
3. Discrepancies Between Inferred and Observed CO32 Changes
The paper highlights a discrepancy between the large changes in [CO32−] inferred from the mass-balance calculations and the relatively small changes observed from foraminiferal proxies. This discrepancy is explained by the significant increases in soft tissue and disequilibrium DIC (dissolved inorganic carbon) that occurred in the deep sea during glacial periods. The larger solubility of CO2 in colder water would have also contributed. Complex Earth system models support this hypothesis, showing increases in soft tissue and disequilibrium DIC comparable to the simulated [CO32−] changes. The combined effect of these additional carbon storage mechanisms, which are not explicitly included in the simplified mass-balance calculations, helps reconcile the difference between model predictions and the actual observations. It highlights the limits of simplified models and the importance of including more detailed biogeochemical processes for accurate representation of the carbon cycle during glacial cycles.
4. Implications for Whole Ocean δ13C and the Terrestrial Biosphere
The discussion explores the implications of the findings for whole-ocean δ13C and the terrestrial biosphere. Changes in shelf burial significantly impacted the global CaCO3:Corg burial ratio, influencing the δ13C of the active carbon inventory. The lower proportion of low-δ13C Corg buried during low sea levels would have led to a decrease in the δ13C of the active carbon pool. This invalidates the simple use of whole-ocean δ13C to reconstruct changes in the terrestrial biosphere. Mass-balance calculations, while uncertain, suggest a potentially larger terrestrial biosphere during the LGM than previously assumed based on a constant-inventory assumption. Using the highest modern Corg burial flux estimates implies a δ13C change exceeding that observed in the deep ocean, suggesting inconsistencies either in the high burial flux estimates or the assumption of a constant terrestrial biosphere during the LGM. The latter could be explained by the possible expansion of peatlands and permafrost carbon storage during glacial periods. The results emphasize the interconnectedness of the ocean and terrestrial carbon cycles.